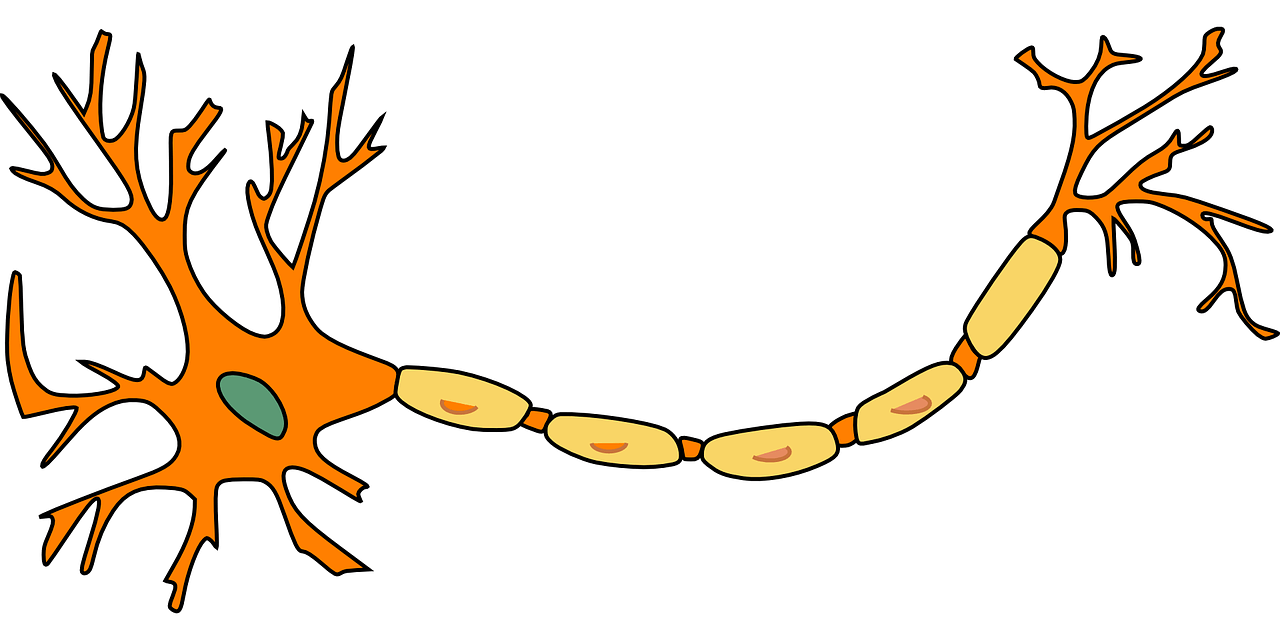
Neurons are the basic organizational units of the brain and nervous system. Neurons form the bulk of all nervous tissue and are what allow nervous tissue to conduct electrical signals that allow parts of the body to communicate with each other. Neurons are the cells that are responsible for receiving sensory input from the outside world, sending motor commands to move parts of the body, forming memories in the brain, and more. Virtually every aspect of our experiential life and engagement with the world is mediated by the activity of neurons.
The human brain is estimated to contain over 100 billion neurons with each neuron having, on average, connections to 1000 other neurons. This vast connectional architecture explains that computational complexity of the human brain. Neurons communicate with each other by generating and conducting electrical signals. The constant electrical signaling is the physical correlate for our thoughts, emotion, feelings, sensory experiences, etc.
Parts Of A Neuron
All neurons share a few basic anatomical features.
Dendrites: Dendrites are the receiving part of the neuron, the part that actually receives the electrical signal from other neurons. Dendrites look like long spindly extensions that branch out of the main body of the cell. In some kinds of neurons, dendrites themselves have further protrusions called dendritic spines that assist in electrical conductivity.
Axon: The axon of a neuron is a long extension of a neuron that actively propagates electrical impulses away from the neuron. Axons work to transduce electrical signals to other neurons, cells, and glands. The swollen end of the axon that connects to the dendrites of another neuron is called the axon terminal. The junction where the axon terminal of one neuron meets the dendrites of another is called a synapse. Axons can range from just a few centimeters to over 3 meters in length. Most axons are covered in a lipid-based substance called myelin in the form of the myelin sheath that facilitates electrical conduction along the axon. Myelin is produced by a special group of cells called Schwann cells.
Several neurological disorders result from degradation of the myelin sheath in the axons. For example, multiple sclerosis is a disorder characterized by the immune system attacking its own myelin sheath and Schwann cell. The demyelination of the axon explains the symptoms of MS; loss of sensory sensation, involuntary muscle control, and cognitive difficulties.
Soma: The soma (cell body) is the central mass of the neuron and contains the cell’s nucleus. The nucleus contains the neuron’s DNA and is the site of RNA transcription and translation which produce proteins necessary for the neuron to function properly. The neuron transports proteins from the soma to the axon and dendrites via the activity of microtubule-associated motor proteins.
Types of Neurons
There are many different kinds of neurons whose morphologies and function vary. Some neurons do not have dendrites, some have 1 dendrite and 1 axon, and some have multiple of each. Neurons can be differentiated based on where they originate, where they connect to, their anatomical structure, and what function they perform in the nervous system.
In general, there are a handful or structural varieties of neurons:
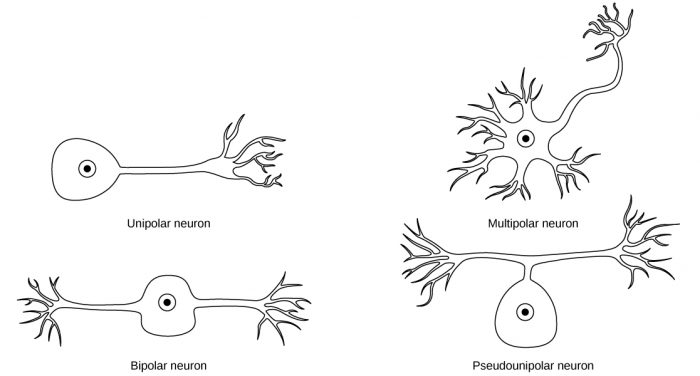
The structural varieties of neurons. Credit: CNX OpenStax Biology via WikiCommons CC-BY 4.0
- Unipolar: Contains only one branching body
- Bipolar: Has 1 axon and 1 dendron
- Multipolar: 1 axon and 2 or more dendrites
- Anaxonic: Axon cannot be distinguished from dendrites
- Pseudounipolar: Single axon/dendrite fiber with a soma protrusion
Dividing and classifying the different kinds of brain cells is a massively complex task. There is currently no consensus about how many kinds of neurons exist in the brain, but scientists have identified 3 major kinds of neurons in the spinal cord: sensory, motor, and interneurons.
Sensory Neurons
Sensory neurons are the ones that are activated by external stimuli in the environment. When you press on your arm, sensory neurons in your skin detect that force, send a signal to the spine which relays it to the brain. producing the sensation of pressure. Sensory neurons can be activated by chemical and physical stimuli that correspond to the different kinds of sensory modalities: sight, sound, smell, heat, force, pressure, orientation, etc.
Motor Neurons
Motor neurons originate from the spinal cord and branch and attach to the muscles, skeleton, organs, and glands in the body. Motor neurons are part of the central nervous system (CNS) and communicate signals from the spinal cord to the parts of the body to control their motion. For example, motor neurons send signals to the muscles in your arms causing them to contract. Motor neurons send electrical signals to your intestines so they move and churn food.
There are two distinct types of motor neurons. Lower motor neurons connect the parts of the boy to the spinal cord. Upper motor neurons connect the spinal cord to the rest of the brain and carry the initial motor signal that makes its way to the body’s extremities.
Interneurons
Interneurons are the “in-between” units that connect distinct neurons in the spinal cord. Interneurons form the connection between sensory and motor neurons. Interneurons can also form independent feedback loops of varying complexity amongst themselves.
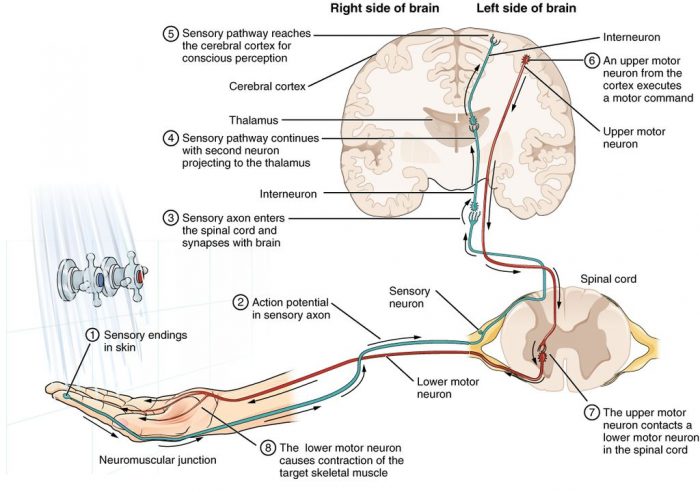
A diagram showing how sensory neurons, motor neurons, and interneurons work to sense and react to hot water. Credit: “Sensory Neuron Test Water” by OpenStax via WikiCommons CC-BY 4.0
How Do Neurons Communicate With Each Other?
We keep saying that neurons work by “communicating” with each other. How, exactly, do neurons do this?
Neurons communicate via electrical events called action potentials. During an action potential event, the cell membrane potential at a specific point on the axon rapidly rises then drops, causing the membrane potential to drop elsewhere along the axon. An action potential event in Neuron A causes the release of neurotransmitters in the synapse that can either excite or inhibit an action potential event in neuron B. Whether or not a particular neuron will undergo an action potential event depends on the total number of excitatory and inhibitory inputs it receives from surrounding neurons. The synchronized excitation, inhibition, and firing of billions of neurons through the body is what constitutes the activity of the nervous system.
How Do Neurons Produce Action Potentials?
All neurons, like all animal cells, are covered by a phospholipid bilayer cell membrane. In general, phospholipid bilayers are poor electrical conductors, but neuron membranes contain special electrically active proteins embedded in their structure. These proteins, called ion channels control the flow of chemical ions into and out of the cell. Changing the concentration of ions in and out of the cell creates an electrochemical gradient across the cell membrane which is the basis for electrical signal transmission between neurons.
Let’s imagine a single neuron that is about to undergo an activation event. In its resting state, the concentration of sodium ions outside the neuron is about 10 times greater than inside the neuron and the concentration of potassium ions if greater inside the cell. In this resting state, the membrane potential is equal to about -70mV, which is called the resting membrane potential.
When neurons receive signals from other neurons, the ion channels in the membrane open letting ions in and out and changing the concentrations. depending on the kind of input (excitatory or inhibitory) the membrane potential will either become more positive (excitatory) or more negative (inhibitory). When the membrane potential hits about -50mV, the sodium channel opens up and the sudden influx of positive cations causes the membrane to rapidly change its potential towards 0. This process is called depolarization.
The concentration gradient of the sodium ion is so strong it actually overshoots depolarization and the membrane potential reaches about +30mV. At this point, different ion channels open up and draw potassium ions out of the cell, causing the membrane potential to go back towards its resting state of -70mV. This process is called repolarization. Again, the neuron overshoots this mark and there is a certain refractory period where the voltage is more negative than -70mV before settling.
These two processes in rapid succession—depolarization and repolarization—constitute the action potential event, which can be visualized by a graph of voltage over time:
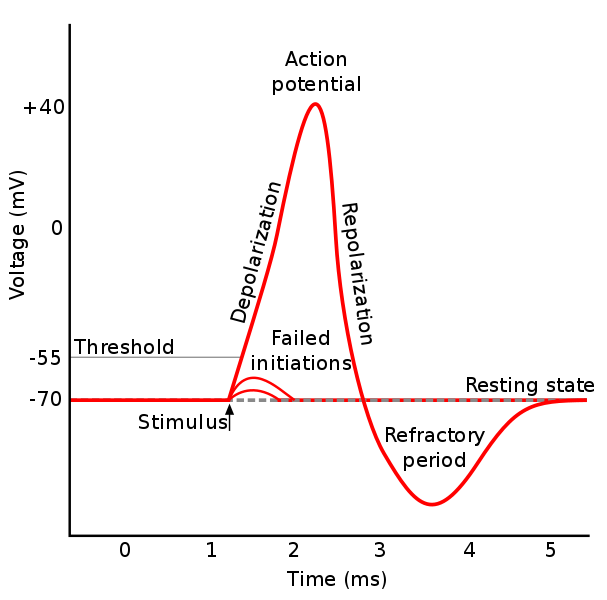
A voltage-time graph showing the action potential. Credit: “Action potential” by Chris73 via WikiCommons CC BY-SA 3.0
Action potential events are often called “spikes” due to the shape the graph takes when voltages are recorded with equipment. The temporal sequence/frequency of spikes is called the “spike train” of the neuron.
Neurons “talk” to each other across the synaptic gap between the axon terminal and the dendrites. When an action potential event is initiated, the signal travels down the axon to the terminal where it releases neurotransmitters into the synaptic cleft, the space between the axon terminal of one neuron and the dendrite of another. These neurotransmitters are picked up by receptors in the dendrites where (depending on the kind of signal) will cause either positive or negative ion channels to open up in the neuron. Synapses can be thought of as converting an electrical signal (action potential) into a chemical signal (neurotransmitter) and then back to an electric signal again in the form of changes to the membrane potential of the terminal neuron.
Key Points/Summary
- Neurons are a type of cell and are the fundamental constituents of the nervous system and brain.
- Neurons take in stimuli and convert them to electrical and chemical signals that are sent to our brain.
- There are 3 major kinds of neurons in the spinal cord: sensory, motor, and interneurons.
- Neurons communicate vie electrical signals produced by action potential events.
- Action potential events are generated by differences in the concentration of ion across neuronal membranes
- When an action potential event occurs, the membrane potential rapidly depolarizes then repolarizes to its resting state.
- Action potential events produce neurotransmitters in the synapse, which are picked up and either excite or inhibit action potential events in other neurons