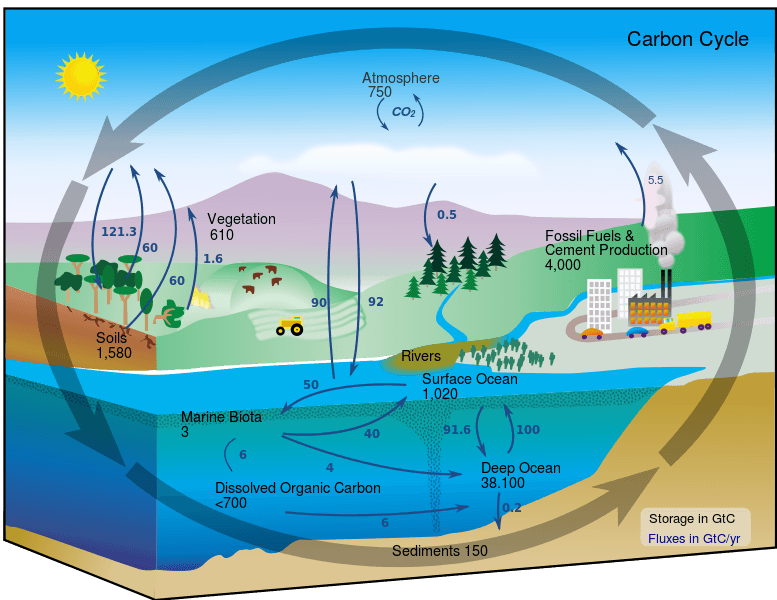
Carbon is quite possibly the most important element required for life. Every living creature requires carbon to function and most organic molecules consist of chains of hydrogen and carbon molecules. About 18% of your body by mass is made entirely of carbon, without which you would have no DNA, cells, or even basic glucose molecules to metabolize for energy. Carbon is so useful for life because of its electron structure. It has 4 valence electrons which allow it to create a virtually endless amount of organic compounds. Carbon is also an important component of many kinds of rocks and minerals.
The carbon cycle refers to the biogeochemical process by which carbon is cycled through the atmosphere, oceans, geological strata, and living systems on earth. The carbon cycle, along with the water, nitrogen, and phosphorus cycles, forms a network of extremely important interactions that are necessary for life as we know it.
Carbon Cycle: A General Overview
The carbon cycle concerns the transfer of carbon between important components of Earth’s systems. These components form the bulk of carbon reserves on the planet. The 4 major components of the carbon cycle are:
- The atmosphere
- Oceans
- Terrestrial biosphere (living systems)
- Geological sources (sediment/ crust and mantle)
Together, these 4 separate systems interact and cycle carbon between each other. The cycle of carbon between these 4 components is facilitated by a wide variety of chemical reactions. Over time, these cycles have developed to maintain a near constant level of carbon in each component. The carbon cycle has been severely offset due to the exponential growth of human carbon emissions in the last 150 years.
Subcycles Of The Carbon Cycle
Generally, the carbon cycle is split between two subcycles; the first concerning the short-term transfer of carbon materials among living organisms and the second focused on the long-term cycling of carbon through geological processes. These two subcycles are separated but interconnected in that they both rely on the same stores of carbon and influence each other.
Biological Carbon Cycle
In the first subcycle (called the biological carbon cycle), carbon in the form of atmospheric carbon dioxide is taken in by photoautotrophs and converted into organic molecules. These organic molecules are consumed by heterotrophs who produce carbon waste products and exhale carbon dioxide back into the soil and atmosphere. The breakdown of organic molecules into their carbon components is called cellular respiration. Decomposers in the environment further break down waste products when they consume dead organisms and plant matter. Every year, about 100,000 to 100 million tons of carbon is cycled through these pathways.
Geological Carbon Cycle
The second cycle (sometimes called the geological carbon cycle) takes much longer to cycle carbon. Atmospheric carbon dioxide dissolves in the oceans and reacts with water molecules to create bicarbonate. Bicarbonate is a major component of many structures of marine animals, such as mollusk shells. When these organisms die, their carbon-containing remains become part of the sediment on the ocean floor. Over time, carbon from these deposits seep into the geological strata and form limestone and other mineral compounds. These carbon deposits seep up through the ground into soil deposits or turn into fossil fuels that humans burn, releasing carbon dioxide into the atmosphere. In general, the geological carbon cycle takes millions of years for materials to cycle through the oceans and Earth’s geological strata.
The biological and geological carbon cycle are related and feed into each other. Carbon deposits from the geological cycle are also taken up by plants and used to photosynthesize. Carbon from the oceans evaporates into the atmosphere, and geological activity like earthquakes, tectonic movements, and volcanic eruptions put carbon back into the atmosphere. Together, the biological and geological carbon cycles make up the overarching carbon cycle on Earth. Without this complex interplay of the two subcycles, carbon would not be available in amounts necessary for life.
Let’s take an in-depth look at each component of the carbon cycle to see exactly how it uptakes and cycles carbon.
Components of the Carbon Cycle
Atmosphere
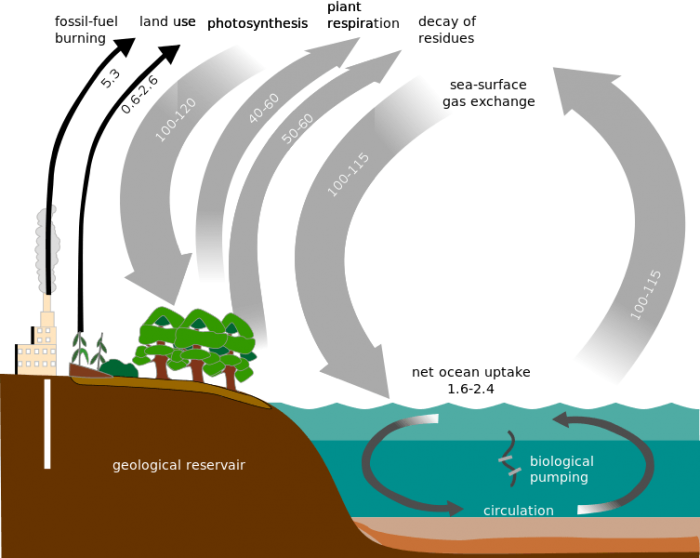
Carbon from the atmosphere is transferred between all major carbon sinks on Earth. Credit: Public Domain
The atmosphere is one of Earth’s largest carbon sinks and contains an estimated 720 gigatons (1 gigaton = 1 billion tons) or carbon. Carbon in the atmosphere exists mostly in the forms of carbon dioxide and methane (CH4). Both of these gases are greenhouse gasses which trap heat, warming the atmosphere. Of the two, carbon dioxide exists in greater proportions and methane has a much shorter lifespan. The amount of carbon dioxide in the atmosphere has nearly doubled in the past 200 years, driven largely by human activity.
Carbon dioxide is removed from the atmosphere mostly through photosynthesis by autotrophs where it enters the terrestrial biosphere. This is the direct source of all organic materials that heterotrophs eat, humans included. Atmospheric carbon is the basic building block of organic molecules. Carbon is cycled back into the atmosphere via the exhalation of carbon-consuming heterotrophs, evaporation from the oceans, and geological activity. The rate at which carbon is cycled through the atmosphere differs greatly depending on a number of factors.
Oceans
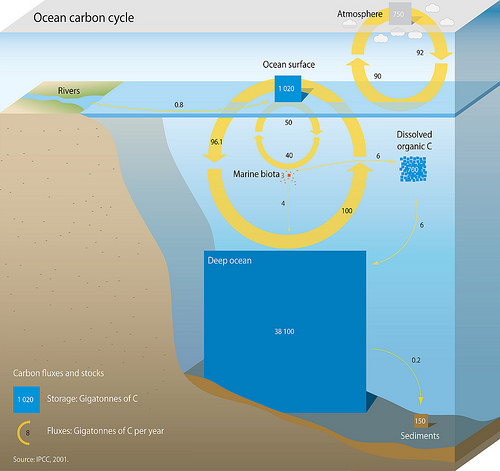
Credit: Public Domain
By itself, the Earth’s oceans are the single largest carbon sink on the planet. At any given time, over 93% of carbon on the planet is contained in the ocean, either dissolved in water or in the form of algae, coral, and animals under the sea. There are three main processes that facilitate the cycling of carbon through the ocean.
The solubility pump involves the uptake of atmospheric carbon dioxide by the oceans. Carbon dioxide in the atmosphere dissolves into the oceans and reacts to form carbonic acid and bicarbonate via the following chemical equation:
CO2 + H2O → H+ + HCO3−
This mechanism also works to control the pH levels of the ocean, keeping them slightly basic.
The second mechanism is known as the carbonate pump. Carbon uptake from the process begins in the form of calcium carbonate (CaCO3) Calcium carbonate is the main constituent of hard shells of marine animals, like mollusks, phytoplankton, and other invertebrates. Calcium ions in the ocean combine with bicarbonate ions which produces calcium carbonate and releases carbon dioxide according to the equation:
Ca2+ + 2HCO3− ⇔ CaCO3 + H2O + CO2
The biological pump refers to the fixing of carbon materials via photosynthetic organisms in the oceans. Like plants on land, marine organisms such as cyanobacteria, algae, and plankton take in carbon dioxide via photosynthesis and create organic molecules that other marine animals rely on for food.
Before the industrial revolution, the oceans were primarily a source for atmospheric carbon. Due to carbon emissions from humans, this relationship has been reversed and now the oceans operate as a sink for atmospheric carbon. Rising ocean temperatures have negatively affected our ocean’s ability to efficiently absorb carbon.
Terrestrial Biosphere
The “terrestrial biosphere” refers to the entirety of land-based ecosystems and living organisms. The majority of carbon in this component is found in forests, contained in the trees and in the soil. Carbon in the biosphere is introduced mainly through the activity of terrestrial photoautotrophs who use it to create organic materials. These materials are cycled through the biosphere via the consumption of plants by heterotrophs. When animals eat plants, their carbon is used to drive cellular respiration which release carbon dioxide back into the atmosphere. In the case of aerobic respiration, methane is produced. Anaerobic respiration produces methane. Dead plant material also settles in the soil where it can remain as “inert” carbon for a long period of time.
Geological Sources
Geological structures are also a major carbon sink and producer of carbon. The majority of carbon activity in the Earth’s crust is driven by plate tectonic movement and geological reactions like chemical weathering. Of the different components of the overall carbon cycle, carbon takes the longest to cycle through geological strata. The main sources of carbon in geological structures are various kinds of carbonate rock, such as limestone. Atmospheric carbon dioxide reacts with exposed silica rock which creates limestone and other carbon-containing rocks. Over time, these rocks dissolve and are carried to the oceans, where they settle on the floors as sediment. As Earth’s tectonic plates move, this sediment is slowly introduced into the Earth’s crust and mantle.
Carbon contained in Earth’s geosphere is released during periods of geological activity. The most obvious example is during the formation of mountains and volcano eruptions. During these processes, large scale plate tectonic activity breaks up carbon-containing rocks, carrying them to the surface and releasing carbon dioxide. Silicate rocks revealed during these processes act as a carbon sink, taking in atmospheric carbon so the cycle can start all over again. Scientists can actually tell quite a bit about the geological history of Earth by examining carbon deposits trapped in geological strata.
Human Effects On The Carbon Cycle
Since the beginning of the industrial age, carbon emissions stemming from human activity has caused large scale shifts in the distribution and cycling of carbon. Fossil fuel consumption directly increases the amounts of atmospheric carbon dioxide and other practices such as deforestation reduce the effectiveness of natural carbon sinks. It is estimated that since the 1950s, almost 60% of new carbon introduced into the atmosphere yearly has been the result of human emissions. Anthropogenic sources of carbon dioxide have disrupted virtually every point in the natural carbon cycle and have caused global temperatures to rise and the oceans to become more acidic and less hospitable to life.