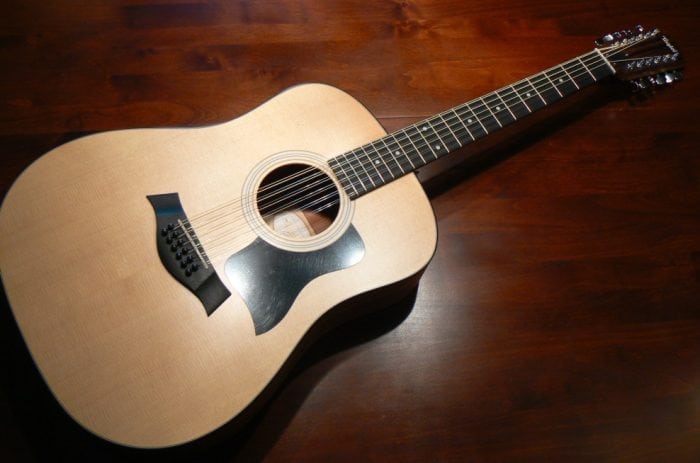
The acoustic microscope is a critical component for a variety of applications including quality control, medical imaging, biomedical sensors, and focused ultrasound surgery, owing to its ability to distinguish objects separated by a small distance.
The notion of an acoustic microscope can be traced back to 1936 when Sokolov proposed a device to provide magnified views of a structure by high-frequency sound waves. However, the implementation was handicapped by contemporary technological restrictions. Despite the rapid development in this area, the current acoustic microscope still suffers from the diffraction limit, since the evanescent waves carrying the subwavelength information of targets decay exponentially with increasing distance, making it hard to achieve super resolutions in real applications.
It was always expected to develop an acoustic magnifying lens that could provide magnified images with subwavelength details of objects, but it was too hard to realize until the start of this decade when the concept of metamaterials was firstly proposed, which opens a new avenue to break the diffraction limit that had dogged scientists for a long time. It had been theoretically proved that ‘perfect metamaterial lens’ was able to recover evanescent waves emitted by the sources in the far fields and hence created images without any distortions. However, the concept was noticeably hampered in the acoustic region by finding dispersion-free and lossless meta-atoms with the refraction index n=-1. An alternative approach to realize the super-resolution is through a hyperlens. However, it is very sensitive to the positions of sources, implying that only objects located close to the circumference of the lens can be properly resolved.
Recently, a new approach to realize acoustic subwavelength imaging has been proposed to address this challenge based on the theory of transformation acoustics. Generally, this method provides a paradigm to implement sound-manipulating devices that were either difficult or impossible to design using other traditional tools. Such transformation could be interpreted in terms of acoustic materials in real space with transformed bulk modulus and mass density values and allowed the space around the objects to be reshaped such that sound can propagate along the prescribed trajectory. A couple of transformation strategies was considered in the design of the magnifying lens to resolve objects gathered closely, which can efficiently transfer subwavelength details from objects to far field.
The fabricated lens is constituted by a core with high refraction index and a concentric shell with gradient refraction index. A flower-shaped element is utilized to serve as the basic unit of the lens, and their dimensions are varied gradually in the radial direction to meet the demand of material parameters. The fabrication is accomplished through three-dimensional printing that is a commonly employed technique in engineering. The measurement results clearly demonstrate the strong ability of the lens to promote the acoustic imaging quality, which achieves a resolution of 0.36λ from 5650 to 6350Hz, making it a potential candidate for industrial applications due to the advantages of easy implementation, low loss, and compact size.
This study, Acoustic Metamaterials: Acoustic Magnifying Lens for Far-Field High Resolution Imaging Based on Transformation Acoustics was recently published in the journal Advanced Materials Technologies.