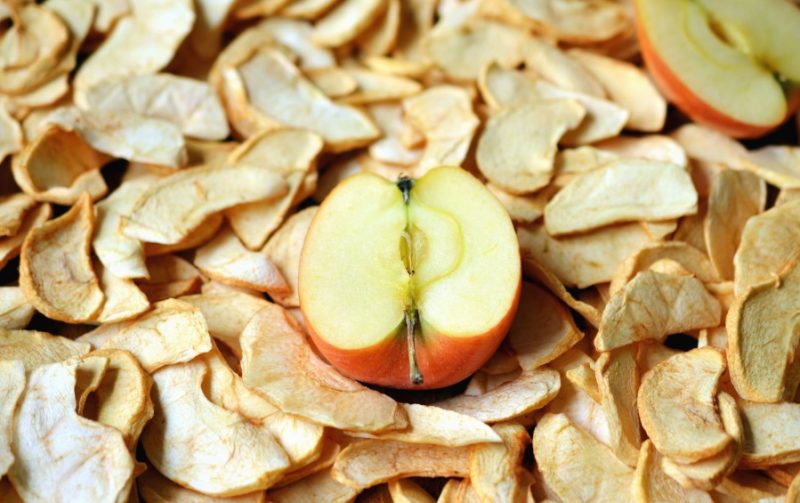
Electrohydrodynamic (EHD) drying is basically a highly efficient way to convectively dry materials. Its unique trait is that airflow is produced locally by corona discharge instead of mechanically by a fan. As such, there are no moving parts nor fan blades.
In order to achieve this, a high voltage difference – several thousands of Volts – is produced between an emitter electrode, for example, a needle or wire, and a grounded collector electrode, typically a plate. As a result, the air around the curved emitter is locally broken down, and ions are formed. These ions are accelerated by the Coulomb force toward the grounded collector. Their subsequent collisions with neutral air molecules induce airflow. This “ionic wind” accelerates the convective drying process of any moist material placed inside the airflow.
As a non-thermal technology, EHD drying is excellent to dehydrate heat-sensitive foods and food ingredients. Typically, dried products include apple, mango, tomato, banana, mushrooms, carrot, meat or seafood.
Why EHD drying?
Compared to standard convective drying, this innovative drying method alters dehydration kinetics, leading to products with different porous microstructure and material properties. For food, EHD drying has been found to reduce drying time and shrinkage, increase rehydration capacity, soften texture, preserve nutritional content, and maintain color and flavor. Ionic airflow production is also argued to consume less energy than a fan.
So what’s the problem?
EHD drying technology has been studied for over two decades now, but so far, no industrial systems are available to our best knowledge. A key hurdle is a limited possibility to upscale the commonly-studied needle-to-plate configurations, where airflow impinges onto the food and collector electrode plate. This configuration leads to drastically reduced moisture removal rates when drying hundreds of products simultaneously, which is required in industrial dryers. Moreover, as evaporation is restricted from the bottom surface, it induces non-uniform drying conditions inside each food product.
What is our approach?
The key to upscaling EHD drying technology lies in novel electrode configurations. However, in order to successfully test and optimize these, insight into the EHD-driven airflow and, at the same time, the dehydration process inside the food products during drying is essential. For this purpose, we developed a mechanistic finite-element model. This continuum model directly couples EHD-generated airflow to the convective moisture removal from food products.
In our study, we evaluated multiple emitter-collector configurations, including a wire-to-mesh geometry. Here, airflow is able to pass alongside the food instead of impinging to it. We demonstrated that we could achieve significantly faster drying rates – roughly up to twofold – when placing food on a mesh instead of a plate. In addition, the food also dried more uniformly on a mesh. This new EHD dryer design is also upscalable without compromising the food drying rates in any way when drying large amounts of products.
In addition to the unique insight in the drying process, the new multiphysics model enabled a swift parametric quantification of EHD process parameters (such as wire radius, distance to the collector electrode, and emitter voltage) and geometrical configurations on the drying rate and the food quality.
What next?
We are currently applying this modeling approach for further optimization of EHD drying technology towards industrial implementation. We are also exploring the various drivers for EHD drying, besides convection, which are linked to the electrical field and ion transport, as we did not yet elucidate their exact contributions.
These findings are described in the article entitled Electrohydrodynamic drying of food: New insights from conjugate modeling, recently published in the Journal of Cleaner Production. This work was conducted by a team including Thijs Defraeye from Empa (Swiss Federal Laboratories for Materials Science and Technology, Laboratory for Biomimetic Membranes and Textiles) and Alex Martynenko from Dalhousie University (Canada).