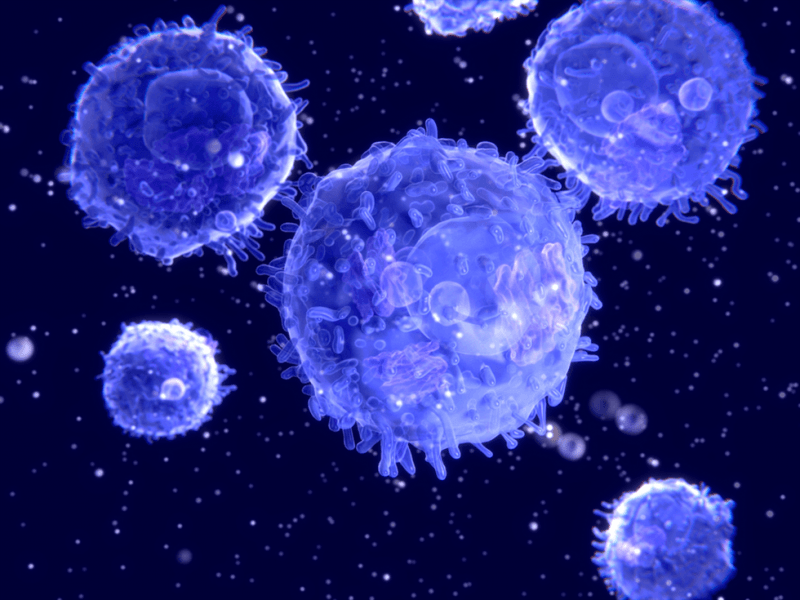
Synthetic biology is challenging our current conception at multiple levels with an unprecedented potential. The aim is the (re)engineering of gene regulatory circuits to end with cells (re)programmed on purpose for biotech/biomed applications (viz., to implement novel or discover natural functions). This is accomplished by introducing suitable synthetic DNA pieces, mostly designed from scratch thanks to the acquired knowledge of molecular biology, in the target cell.
But, “such engineering needs to be carried out through the combination of control mechanisms at different points of the genetic information flow and the application of ab initio sequence-to-function models able to predict dynamic gene expression,” says Guillermo Rodrigo, a researcher in the Spanish Research Council and leader of a recent article of synthetic biology. This will allow a maximal integration capacity of signals in the circuits and a full design automation of them with computational models. In this regard, RNA appears to be a suitable substrate from which to achieve this goal.
RNA is a highly-programmable macromolecule able to deploy a wide variety of functions in the cell. Because of that, it captured the attention of pioneer researchers in the field to develop synthetic riboregulators (i.e., RNA molecules with regulatory ability). In recent years, we have witnessed a renaissance of RNA synthetic biology through the development of novel riboregulators, the incorporation of further RNA elements, such as ribozymes (RNAs with catalytic activity), and the maturity of CRISPR-based regulatory methods. All this is allowing the construction of functional genetic circuits beyond single regulations.
Genetic circuits (i.e., the way that genes “talk” to each other within the cell) behave in an equivalent way to electronic circuits in order to perform logic operations. Hence, those circuits can be described by means of diagrams similar to those used in electronics, with nodes representing genes and arrows indicating regulations (of activation or repression). In Boolean terms, the expression of a given gene alternates between 1 (on) and 0 (off) as the input signals propagate through the circuit.
Recently, Rosado et al. have succeeded in programming genetically a bacterial cell with regulatory RNAs in order to perform arithmetic calculations. “We programmed a bacterium to work as a calculator able to make sums,” says Rodrigo. The system, which runs in a single living cell (a bacterium), receives two input signals, processes them, and then generates two possible output responses. Isopropyl β-D-1-thiogalactopyranoside (IPTG) and anhydrotetracycline (aTc) are the two molecules that work as input signals, while the expressions of a superfolder green fluorescent protein (sfGFP) and a monomeric red fluorescent protein (mRFP1) constitute the output responses.
The key point is considering that one molecule codes for one bit of information in binary code (0 if there is no molecule in the medium, 1 if the molecule is present at a sufficient concentration). When there is no IPTG or aTc in the medium, the bacterium is not fluorescent (in Boolean terms, this corresponds to 0 + 0 = 00). However, when there is only IPTG or only aTc, the circuit is able to process this information and, as a result, the bacterium is turned red fluorescent (this corresponds to 0 + 1 = 01 or 1 + 0 = 01, assuming that mRFP1 codes for the byte on the right and sfGFP for the byte on the left in the output response). Finally, when both IPTG and aTc are present, the circuit processes this new information and then the bacterium is turned green fluorescent (this corresponds to 1 + 1 = 10).
Of course, this is a first step regarding the engineering of synthetic gene circuits based on regulatory RNA, which significantly adds to previous developments, mostly based on regulatory proteins. But this should be applied in a real context to fully grasp its potential. For instance, Rodrigo argues that, “if properly remodeled to be able to read new molecular signals (e.g., by changing the promoters that are in charge of expressing the designer riboregulators), this system might be deployed in the gut of a model animal (a worm or a mouse) to work as a smart probiotic to discriminate pathological states.”
Certainly, the ability to process environmental information and generate complex responses is one of the strengths of using living cells as therapeutic and/or diagnostic agents in the body. It is treating biology with biology, beyond chemistry. The implementation of genetic circuits that allow cells to work like computers (i.e., calculators, counters, memories, etc.) is then instrumental for these goals. This scientific and technological revolution is still doing its first steps but promises to shift several paradigms in the years to come.
These findings are described in the article entitled Binary addition in a living cell based on riboregulation, recently published in the journal PLoS Genetics. This work was directed by Guillermo Rodrigo in the Spanish Research Council (in Valencia) and was mainly funded by the BBVA Foundation with a Leonardo fellowship.