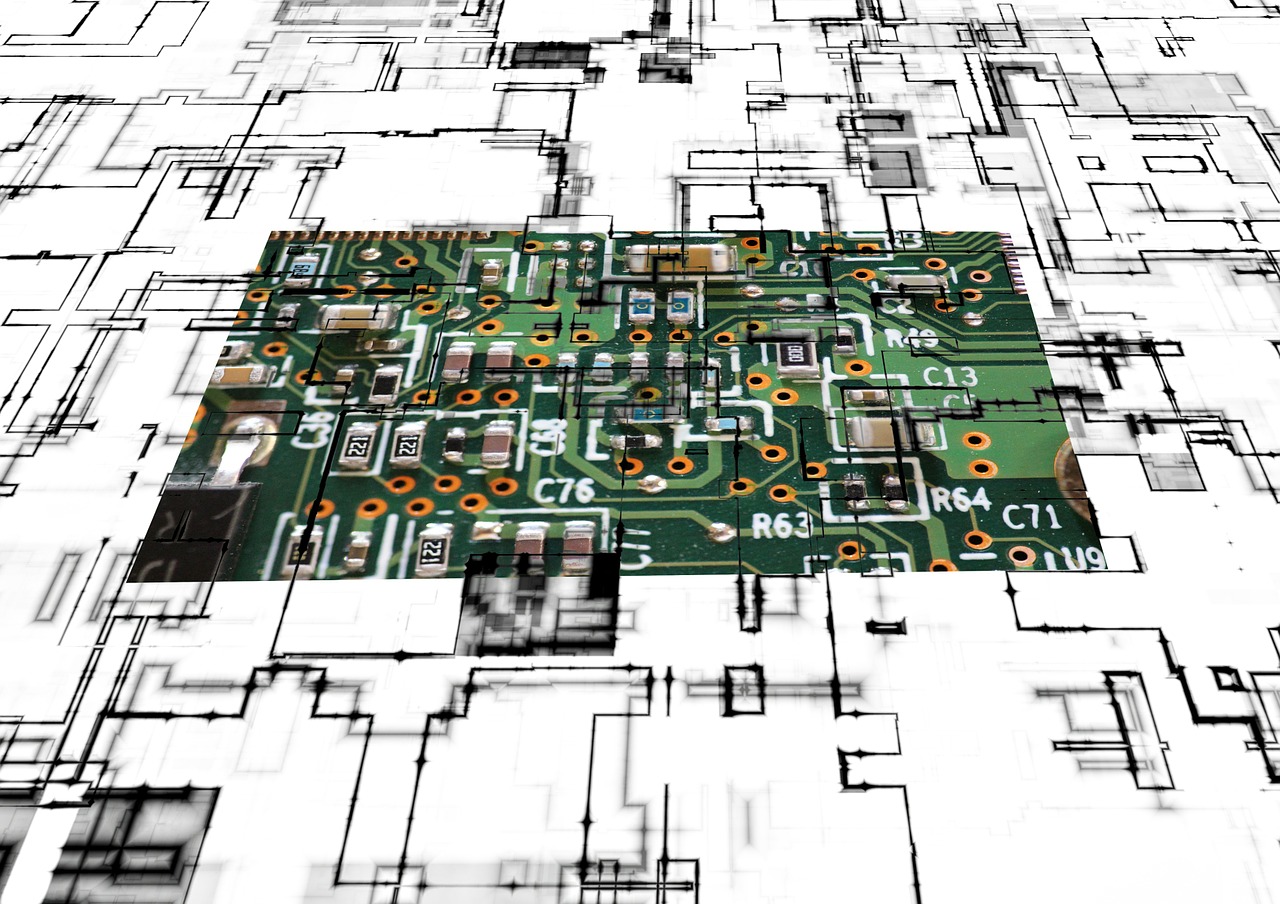
Since the 1920s, scientists have theorized ways to exploit the properties of quantum systems for communication purposes. By utilizing the strange properties of quantum entities and phenomena, like superpositions and entanglement, quantum communication channels could create genuinely unbreakable encryption protocols and provide computing power vastly superior to traditional computing methods.
Now, a team of researchers has taken a significant step toward making the use of quantum communications a practical reality.
In an article published October 1st in Nature, a group of scientists from the University of Groningen in the Netherlands reports that they have developed a reliable method to create entangled pairs of quantum particles at wavelengths close to those that are used by standard telecom providers. The new method relies on exploiting the structural defects of the semiconductor silicon carbide in optical fibers to produce qubits of information that can be transmitted via ordinary communication channels.
Previous attempts at communicating using quantum systems have shown success, but most existing setups require custom hardware, as the produced qubits emit photons at wavelengths outside the range of that used standard optical channels. The discovery of this method is a significant step towards integrating quantum communications into everyday communication systems.
Qubits And Semiconductors
Standard digital computing systems store information in registers called “bits.” In digital computers, a bit can only take on one of two values; either a 1 or a 0 (hence the term binary). Quantum computing systems use “qubits,” a generalization of the classical bit. Qubits can take on three states, 1, 0, or a superposition of both 1 and 0. Essentially, a classical computer bit can exist in only one state at one time while a qubit can exist in multiple states at the same time. The most interesting property of a qubit is that it can store arbitrarily large amounts of classical information, so a quantum computer theoretically could perform tasks that would be impossible or take an extremely long time on a regular digital computer.
To produce a qubit, first, the system has to make an entangled pair of photons. Two entangled particles will have certain values correlated no matter how far apart they are separated, so a measurement on one will instantaneously give you information about the state of the other particle. So, in order for a quantum communication channel to work, there needs to be a reliable way to create entangled pairs of particles. Additionally, these superpositions must be sufficiently isolated from the surrounding environment, as any small disturbance can decohere the superposition into a classical state.
It is known that various transition-metal impurities in semiconductors will emit photons when struck by light. These impurities are known as “color-centers” and can affect the behavior of light. When light is shined on these impurities, excited electrons will jump to a higher energy state. When they fall back to their ground state, the electrons will emit the excess energy as a photon. For a material like silicon carbide with impurities of the metal molybdenum, the photons are emitted at an infrared wavelength close to that used in standard telecom communications channels.
With this information in mind, the team began constructing their system. By using a procedure known as “coherent population trapping,” they were able to create superpositions of electrons in the color-centers of samples of silicon carbide with molybdenum impurities. These superpositions of electrons represented the qubits of information as entangled electrons will always have their spin values correlate. Using magnetic fields, the team was able to align the superpositions in whatever direction they desired. According to Ph.D. student Carmem Gilardoni, one of the researchers credited on the paper, “If you apply a magnetic field, the spins align either parallel or anti-parallel to the magnetic field. The interesting thing is that as a result, the ground state for electrons with spin up or spin down is slightly different.” Shining light on these electrons will make them fall back into one of two ground states and emit an entangled pair of photons.
After some initial attempts, they managed to produce stable and long lasting superpositions at the color centers. The created superpositions showed optical life-cycles of 60 nanoseconds; long enough to extract useful information out of the quantum system. Most importantly, the entangled photons were emitted at a wavelength of ~1100 nm. Traditional optical communications channels use infrared wavelengths of ~1,300-1,500 nm and given the massive amount of knowledge of the effects of transition-metal impurities in semiconductors on the behavior of light, the team is confident that they can fine-tune their procedure to create photon pairs that are emitted at wavelengths that fall comfortably within those used in standard optical channels.
One potential use of this technology would be to create genuinely unbreakable encryptions on communication channels. Superpositions are finicky entities and any disturbance can destroy a superposition by collapsing it into a definite state. If a person attempts to tap into a quantum communication channel to listen in on someone’s conversations, their external interaction with the channel will cause the quantum state to collapse. The result is that it is impossible to eavesdrop on two people who are communicating using a quantum channel, as any attempt to tap into the channel from the outside will decohere the state and destroy the original information.
Other applications of quantum computing include an internet with unparalleled speeds and running simulations of quantum systems. Information stored in qubits can be accessed at dazzling speeds and a single qubit can hold infinitely more information than a classical bit. Though still in its infancy, the potential applications of this technology would completely change the face of modern technology as quantum communication channels could be easily integrated into existing communications hardware, ensuring that the benefits of quantum information systems would be readily available to as many people as possible.