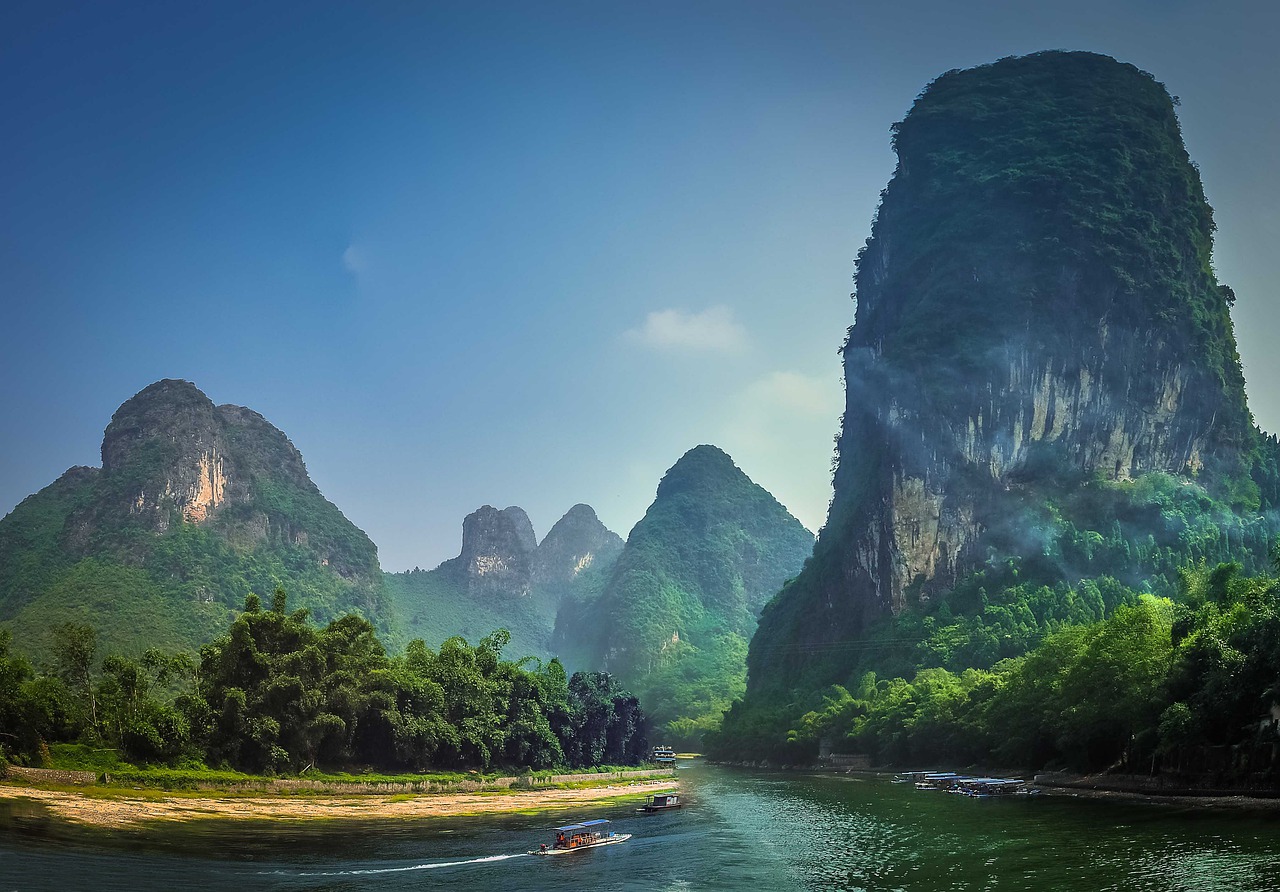
Water pollution is a serious problem in China. Researchers used to not pay attention to surface water, but in recent years, a growing number of studies have been devoted to groundwater pollution, particularly in northern China.
As part of its recent “war on pollution,” the Chinese central government issued a major policy on water pollution control in 2015. The plan aims to address once and for all the chronic problems of water quality, and because of this policy, a huge number of field sampling and analytical campaigns were carried out (Figure 1). In addition to agricultural pollution and domestic wastewater, in many areas, pollution comes from industrial activities.
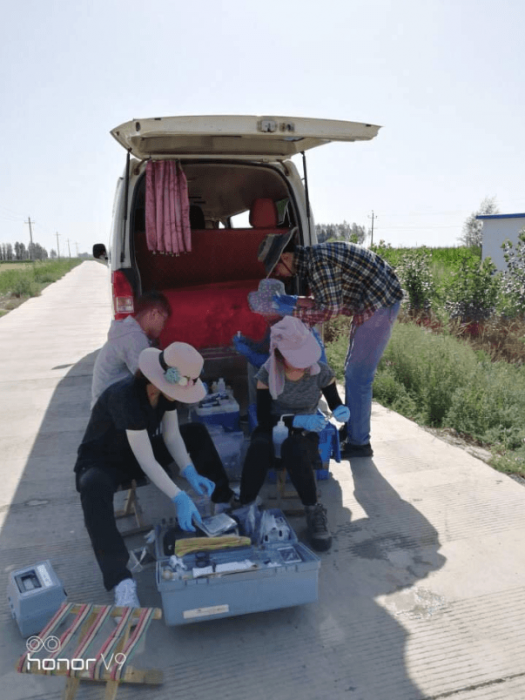
Fig 1: Photograph of the field campaign work in the North China Plain. Courtesy of Haiyan Liu.
The quick expansion of advanced technologies has been accompanied by a huge release of rare earth elements (REEs) into the environment. Natural water has been found to contain REEs (i.e., gadolinium in magnetic resonance imaging contrast agents) at values far above its geological background. In China, REEs have been defined as one of the major emerging contaminants since the 1990s. In the past decades, due to their long-distance transport, bioaccumulation, and toxicity, anomalously high REE concentrations have been commonly detected in water resources. This REE demand leads to an accentuated environmental discharge, posing a potential risk to human health and the ecological system. Therefore, a better understanding of the fate of REEs and their transport through the critical zones (soil, water air…) are important topics for protecting human health and sustaining environmental policy-making.
Over the last three decades, mauch investigation has been performed on REE behavior in groundwater. REE mobilization and transport are mainly driven by reactive solids. Mechanisms such as co-precipitation with secondary minerals, sorption onto iron and manganese oxides, and amorphous iron and manganese precipitation sequestrate and fractionate aqueous REE from solution. It results in low REE concentrations (e.g., from 10−9 to 10-14 mol/L) in circumneutral pH groundwater.
Indeed, in better-constrained interactions with iron oxides, scavenging experiments have shown that scavenged REE increased from about 10% at pH below five to more than 90% at a pH above six in the presence of dissolved iron oxyhydroxide. This outcome contrasts with REE behavior in the absence of iron oxyhydroxides, where scavenged REEs were below 45%. Dissolved carbonate ion has also been shown to influence the solution complexation. A better understanding of geochemical REE behavior requires more widespread and quantitative evidence on REE surface and solution complexation mechanisms.
Many studies have been dedicated to a better understanding REE surface complexation with iron oxides, while less effort has been made toward the quantitative study of REE surface complexation with manganese oxides. The limited existing information suggested that REE binding with manganese oxides could be described using a simple surface complexation model. The model was lately applied to a field study on REE fractionation and mobility in groundwater. Results highlight that REEs show a greater affinity for manganese oxides as compared to iron oxides. Complexation with both of these oxides, however, could be a net aqueous REE sink.
REE sorptive behavior shows a preferential uptake by manganese oxides of light REE (LREE) relative to heavy REE (HREE). Such fractionation trend results in an LREE enrichment in the solid phase. Moreover, dissolved carbonate complexation effect on REE sorption kinetics as well as their fractionation quantified by LREE/HREE ratio should be considered. Indeed, a greater REE fractionation was initially observed on manganese oxides compared to that on iron oxides in the presence of carbonate ligands. More recently, REE complexation with hydrous manganese oxyhydroxides was quantitatively determined using simple surface complexation modeling. The results show that LREE bound onto hydrous manganese oxyhydroxides increased with increasing pH and ionic strength.
Little work has been performed on REE speciation and transport along groundwater flow paths considering REE complexation to hydrous manganese oxyhydroxides, in spite of abundant research published regarding REE behavior along groundwater flow paths or transects. We have already tried to quantify REE binding to iron oxide (see Liu et al., 2017) and manganese oxide in groundwater (see Liu et al., 2018), where we calculated REE speciation in a metal-ligand groundwater system over a range of pH from 4 to 12 by considering various concentrations. The latter study compared the impact of manganese to iron oxides on REE speciation in groundwater along a flow path.
Frequently, manganese has been used as a redox-sensitive component for qualitative evaluation of processes controlling REE mobilization. However, it is essential to quantitatively describe REE binding to manganese oxyhydroxides. Therefore, the objectives of the present study were to (i) identify the roles of hydrous manganese oxyhydroxides in REE transport in groundwater flow path; (ii) to quantitatively define REE surface complexation reactions with hydrous manganese oxyhydroxides from different groundwaters; and (iii) to evaluate the impact of hydrous manganese oxyhydroxides concentrations on REE behavior.
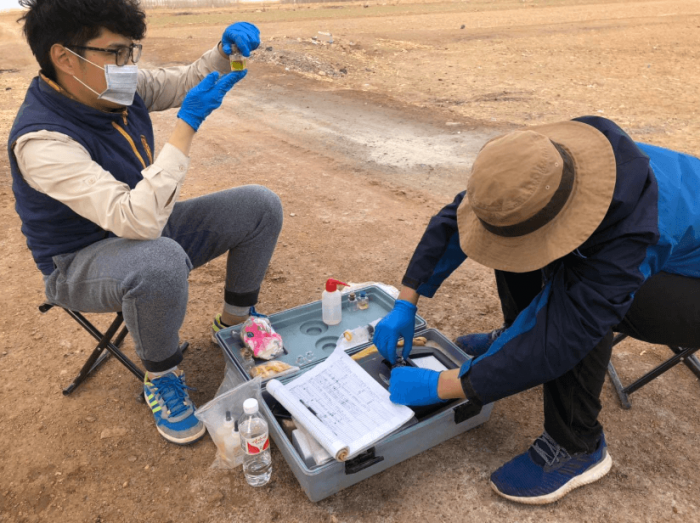
Fig 2: Photograph of the field campaign work in the North China Plain. Courtesy of Haiyan Liu.
In the present project, groundwater was sampled along a flow path in the North China Plain (Figure 2). The main objective was to determine REE behavior linked to surface complexation to hydrous manganese oxyhydroxides by a combined modeling and field study approach. Results showed that the proportion of neodymium (i.e. one of the REEs) complexed by hydrous manganese oxyhydroxides ranges from less than 1% up to 99%. The amount of complexed REEs increases along the flow path. REE bound to hydrous manganese oxyhydroxides exhibits decreasing trends with each increasing atomic number. The process was determined to be independent of pH, hydrous manganese oxyhydroxide’s content, and metal loading.
This finding further demonstrates hydrous manganese oxyhydroxides-REE complexation plays a key role in the transport of REEs in groundwater through preferential scavenging of LREE over HREE. Nevertheless, carbonate ligands appear to be robust competitors in reducing the amount of REE sorbed to hydrous manganese oxyhydroxides when a solution’s pH rises above 8. Assuming that half of Mn concentration occurs as hydrous manganese oxyhydroxides, the amount of complexed REE was predicted to show a more marked decrease in LREE compared to that of HREE.