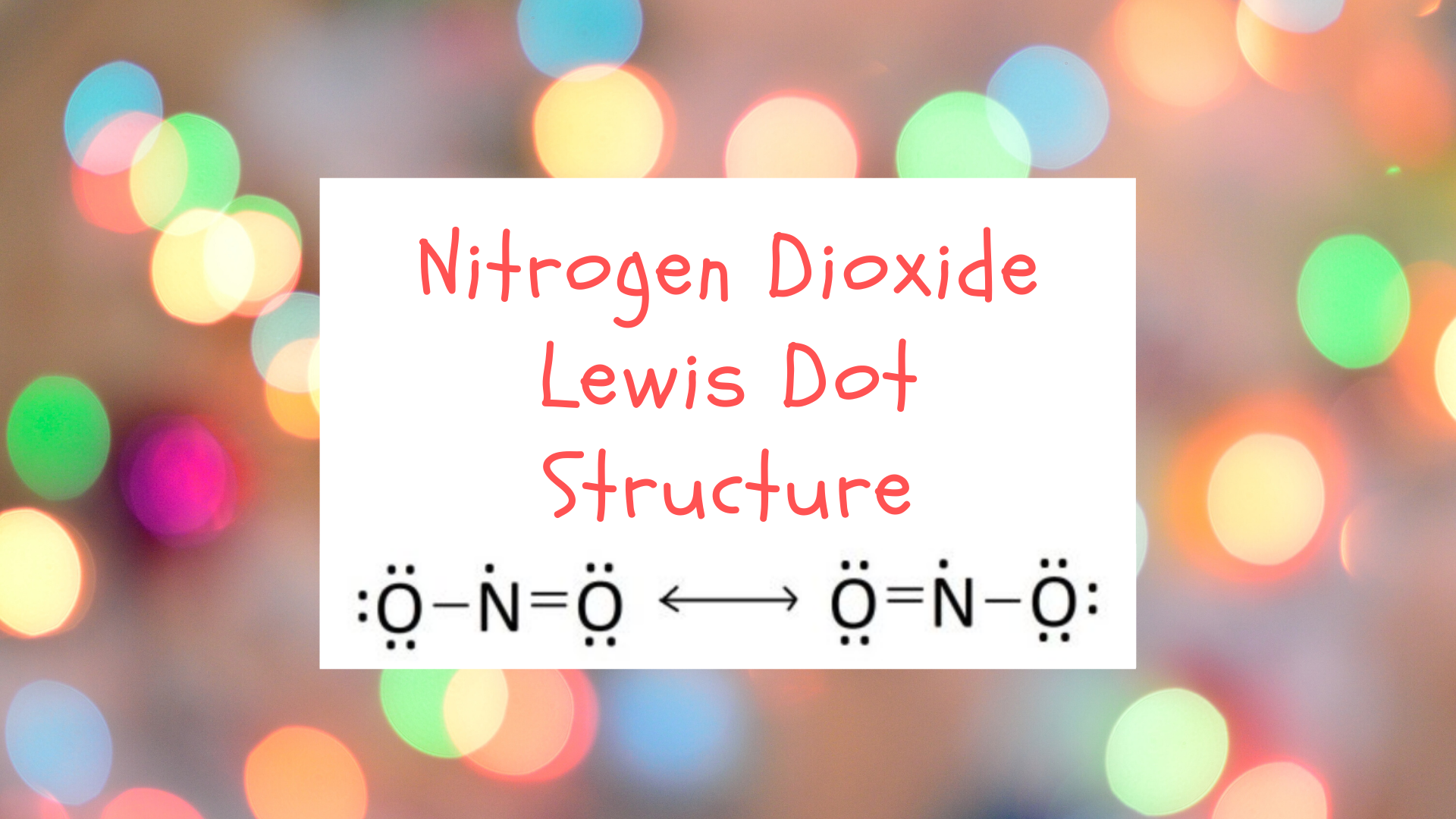
Nitrogen Dioxide (NO2) is a covalent compound that is composed of a central nitrogen atom single bonded to an oxygen atom and a double bond with another oxygen atom. At room temperatures, nitrogen dioxide is a reddish-brown gas that has a density of 1.8 g/dm3. It is slightly toxic to humans, on account of its tendency to react in the human body and produce reactive species of nitrogen and oxygen, which can damage internal structures.
“A small bubble of air remained unabsorbed… if there is any part of the phlogisticated air [nitrogen] of our atmosphere which differs from the rest, and cannot be reduced to nitrous acid, we may safely conclude it is not more than 1/120 part of the whole.” — Henry Cavendish
Nitrogen dioxide does not have a single Lewis structure on account of its relatively strange electron configuration. The location of the double bond changes over time, meaning that at any point, either of the oxygen atoms could have a double bond with the nitrogen atom. As such, nitrogen dioxide is represented by the resonance Lewis structure:
Nitrogen dioxide requires a resonance Lewis structure because its electron configuration constantly oscillates between the two forms. The “true” electron configuration of nitrogen dioxide is considered to be some average of the two resonance structures given above. The Lewis structure of nitrogen dioxide is also interesting because there is a single unpaired valence electron on the central nitrogen atom. Compounds with unpaired electrons are sometimes referred to as “free radicals.” This unpaired electron explains nitrogen dioxide’s reactive behavior as it has a strong desire to fill this open electron spot.
Let’s take a step back and go over the rules for drawing a Lewis structure. We will go step-by-step to see how we can construct a Lewis structure for most main-group compounds, nitrogen dioxide included.
Lewis Structures: The Basics
In a nutshell, a Lewis structure is a pictorial representation of the atomic structure and electron configuration of an atom or a compound. Single atoms are represented by their unique chemical symbol, electrons are represented as single dots, and shared pairs of electrons are represented by a single dash (−) for a single pair, a double bar (=) for a double pair, and a triple bar (≡) for a triple pair.
“Every chemical substance, whether natural or artificial, falls into one of two major categories, according to the spatial characteristic of its form. The distinction is between those substances that have a plane of symmetry and those that do not. The former belong to the mineral, the latter to the living world.” — Louis Pasteur
The purpose of a Lewis structure is to see how the electrons are arranged in an atom or compound. Lewis structures are based on the octet rule—the empirical observation that atoms tend to form bonds until they have a complete valence shell of 8 electrons. The only exception to the octet rule is hydrogen, which will only form bonds until it has 2 valence electrons.
Valence electrons are represented as pairs of dots, where each dot represents a single electron. Atoms form covalent bonds by sharing their valence electrons with other atoms. For example, a single chlorine atom has 7 valence electrons; 3 pairs and one free electron. Two chlorine atoms will share their unpaired electron so that each atom has a full octet of electrons, forming a chlorine molecule (Cl2). In general, this is how covalent bonds work. Atoms will share valence electrons until each atom has a full octet. If all the valence electrons are paired, but an atom still does not have a full octet, electron pairs will move to form double and triple bonds. The total amount of electrons in a Lewis structure is equal to the sum of the number of valence electrons of the individual atoms.
Lewis structures tell you about the atomic arrangement and electron distribution of an atom or compound. While Lewis structures alone do not give explicit information about the 3-D geometry of a molecule, the rules for writing Lewis structures can be combined with rules governing molecular geometry to predict the shape a compound will have.
Rules for Making Lewis Structures
Let’s go through the rules for making Lewis structure, using nitrogen dioxide as our test example.
Step 1. Determine the total amount of valence electrons.
The first step is to figure out how many electrons you diagram should have. The total number of electrons in a Lewis structure should be equal to the sum of the valence electrons of each individual atom. The number of valence electrons of an element can be determined by looking at their group number on the periodic table. In general, groups 1 and 2 elements have 1 and 2 valence electrons, respectively. Group 13-18 elements have 3, 4, 5, 6, 7, and 8 valence electrons respectively. Group 3-12 elements are the transition metals which can have different amounts of valence electrons
In our case, nitrogen dioxide is composed of 1 nitrogen atom and two oxygen atoms. Nitrogen is a group 15 element and so has 5 valence electrons, while group 16 oxygen has 6 valence electrons. There are two oxygen atoms, so the total amount of valence electrons in our diagram is:
5(1) + 6(2) = 17 electrons
Our diagram should have 17 electrons in total.
Step 2: Sketch the atomic structure of the compound
Now that we have the number of valence electrons, we can begin constructing the diagram. If the compound is diatomic (two atoms) the structure is easy; the atoms can be placed side by side. In the case of compounds with three or more atoms, there is normally a central atom that shares multiple bonds with terminal atoms. In general, for triatomic or grater compounds, the central atom is the least electronegative element.
In our case, we have a triatomic compound so our structure most likely will have a central atom bonded to multiple terminal atoms. Nitrogen is less electronegative than oxygen (3.04<3.44), so nitrogen is our central. Placing the symbols give us:
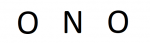
Credit: Author
Step 3: Place pairs of electrons so that every atom has at least one single bond
Next up we can start placing electrons in our model. First, we go through and place a single bond between each atom. Every single bond counts for 2 electrons, so we subtract those electrons from out total amount to get how many we have left to place.
In our case, we place two single bonds, one between each atom, which looks like:
Since we placed 2 pairs, we placed 4 electrons in total. We now have 17-4 = 13 more electrons to place.
“Modern chemistry, with its far-reaching generalizations and hypotheses, is a fine example of how far the human mind can go in exploring the unknown beyond the limits of senses.” — Horace G. Deming
Step 4: Place electron pairs, starting with the terminal atoms, until each has a full octet.
Next, we place the remaining electrons. Beginning with the terminal atoms, fill in dots until each atom has a total of 8 valence electrons. If you have any leftover atoms, place them on the central atom in pairs or as lone electrons.
Beginning with the terminal oxygens first, we place 6 electrons around each so that they have a full octet. Any leftover electrons we place on the nitrogen atom. Adding 6 electrons to each oxygen atoms is 12 total, so we place the remaining single electron on the nitrogen atom:
After placing these 13 electrons, we now have 13-13 = 0 electrons left to place. But, we are not done yet because our central atom still does not have a full octet, nitrogen currently only has 5 electrons; 2 pairs and a single unpaired electron.
Step 5: Move electron pairs to form double and triple bonds until each atom has an octet, or is as close as it can get to an octet.
If all the electrons have been placed and some atoms still do not have a full octet, then compounds will form double and triple bonds to make sure every atom gets as close to 8 electrons as possible. Simply move electron pairs from terminal atoms to make double and triple bonds.
In our case, we have all of our electrons placed buy nitrogen only has 5 valence electrons. Moving an unbonded electron pair from one of the oxygen atoms creates a double bond with nitrogen, giving it 7 electrons. Moving any more electron pair would give nitrogen more than 8 electrons, so we have gone as far as we can and our Lewis structure should look like this:
Resonance Structures
In the last step of drawing our Lewis diagram, we needed to choose an electron pair to move to make a double bond. We picked the left oxygen atom, but couldn’t we have picked the right oxygen atom to get something like this?:
The answer is yes, this is also a valid Lewis structure for nitrogen dioxide. However, this structure is obviously different for the previous; the double bond is on the right instead of the left. If both Lewis structures are legitimate, then which one is the “actual” Lewis structure of nitrogen dioxide? The answer is: both.
In cases where there is more than one legitimate Lewis structure for a compound, the entire Lewis structure is represented as an average of the multiple structures. These structures are known as resonance structures and they are used for compounds whose electron configuration cannot be completely represented by a single unique Lewis diagram. A resonance structure for our two diagrams for nitrogen dioxide looks like:
The “actual” structure of nitrogen dioxide is interpreted as some combination of the two diagrams. Resonance structures are possible because, for some compounds, electron pairs are delocalized and oscillate between one configuration and another. Resonance structures are required because some molecules’ atomic configurations cannot be accurately captured with a single Lewis structure.
Limitations Of Lewis Structures
Following the rules of Lewis structure should allow you to construct a Lewis structure for most compounds made from main group elements in the s- and p-blocks of the periodic table. Some families of elements do not always obey the rules for making Lewis structures. Transition metals, for instance, often do not follow the octet rule and can bond and get up to 12 valence electrons. The further down the periodic table one goes, the less and less the basic rules for Lewis structures apply due to the extremely heavy nuclei and strong electromagnetic properties of large elements.