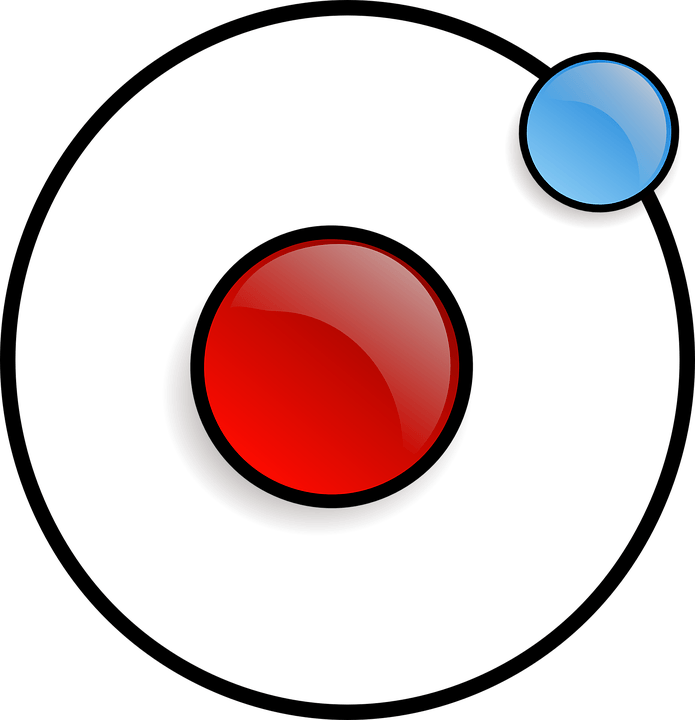
An electron is a subatomic particle that has a fundamental unit of negative charge e−. The electron is one of the most important particles in the Standard Model of particle physics and is one of the 3 primary constituents (along with the proton and neutron) of ordinary baryonic matter. Electrons are considered elementary particles because they do not appear to have any smaller parts or substructures.
The most precise recorded measurements of the electron gives it a mass of 0.000548579909067 amu, which is equal to 9.109382902843941771×10-31 kg.1 This quantity is about equal to 1/1836th the mass of a proton. As such, the electron is the least massive of the normal subatomic particles by far.
Electrons are directly responsible for the bulk of a substance’s physical and chemical behavior. Chemical bonding is determined by electron activity, molecular shape is determined by electrostatic potential, color is determined by the absorption of light by electrons, and the phase behavior of substances (freezing, melting, sublimation, etc. depends on intermolecular electrostatic interactions. Additionally, electrons (along with positively charged particles like protons) are responsible for the phenomena of electromagnetism.
Properties Of Electrons
Electrons are considered elementary particles, meaning that they do not have any proper parts or substructures. Each electron has an electric charge of about −1.602×10−19 coulombs. The charge possessed by the electron is the smallest possible negative unit of electric charge, so its value is often called the elementary unit of charge. This charge is exactly equal and opposite to the charge of a single proton.
In addition to charge, each electron has a property called spin. Every electron has an intrinsic spin of 1/2. The spin of an electron determines the strength of the magnetic moment it produces, and how the electron is deflected in an electromagnetic field. Spin is sometimes likened to the classical property of angular momentum, as in classical physics, a charged body rotating on its own axis will produce a magnetic field. In actuality, this analogy is inadequate. Electrons are not tiny spherical bodies that rotate on their own axis. They are point particles and unlike the angular momentum of classical bodies, which is a continuous value, electron spin always comes in discrete quantized values. Spin should instead be seen as an intrinsic property of electrons, unique to the quantum realm.
Like all particles, electrons exhibit wave-like properties. An electron can be represented by a mathematical function called a wave function (ψ) that describes the evolution of the position and momentum of the electron over time. Squaring the wave function gives a probability density that shows the likelihood of finding an electron at a given location when making a measurement.
Electrons In Atoms
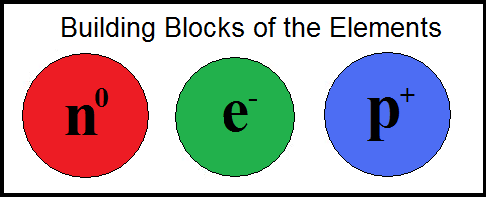
The basic building blocks of ordinary matter and their respective electric charges. Credit: “neutron electron proton” D Larson via WikiCommons CC BY-SA 3.0
Most traditional understanding of electrons comes from our observations of them in atoms. Electrons bond to nuclei containing protons and neutrons due to electrostatic attraction. In general, for a neutral atom, there are equal amounts of protons and electrons. A normal atom of carbon has 6 protons and 6 electrons. The charges of the protons and electrons cancel out, and the entire atom is electrically neutral. Atoms can gain or lose electrons to become an ion. Gaining electrons creates negatively charged ions called anions. Losing electrons creates positively charged ions called cations.
In general, electrons bonded to atoms can only exist in certain predefined states. These states are called atomic orbitals. Orbitals have a predefined shape that determines that qualities of electrons that reside there. Most importantly, each orbital has a definite amount of states and no two electrons can occupy the same state at the same time. This is known as the Pauli exclusion principle, which states that no two electrons can be in the same place at the same time with the same energy. If two electrons exist in the same state, they must have opposite spin numbers of +1/2 and −1/2.
Atoms form chemical bonds by either removing electrons and attracting the produced ion, or by jointly sharing electrons. Compounds formed in the former way are called ionic while compound formed in the latter way is called covalent.
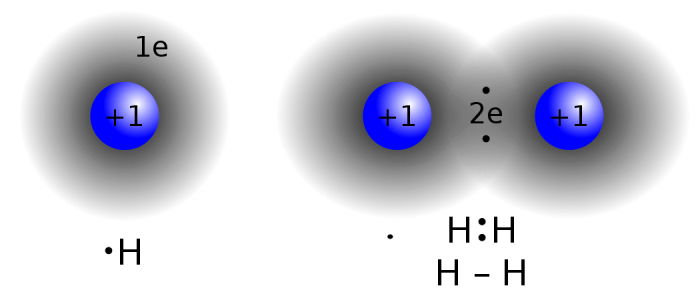
A covalent bond between 2 hydrogen atoms. Credit: “Covalent bond hydrogen” Jacek via WikiCommons CC BY-SA 3.0
When atoms share electrons, they do so by combining their atomic orbitals to create molecular orbitals. When atomic orbitals combine, the molecular orbital takes on a distinct shape that is a combination of the two atomic orbitals. Similar to how waves in a pond will interfere and create new patterns of waves, the standing waves of electron orbitals combine to create a new stable shape.
The bonding behavior of elements is determined by their outermost electrons, called valence electrons. Most elements will form chemical bonds until they achieve a full outer shell of 8 electrons. the tendency for elements to fill their outer shells with 8 electrons is called the octet rule. The octet rule, in conjunction with the rule governing electronegativity, can be used to predict the composition of most main group compounds.
In addition to determining the chemical bonding behavior of elements and compounds, electrons also determine a number of their physical properties. Most obviously, electrons are responsible for giving objects their color. When light hits an object, the photons in light excites the electrons, causing them to go into a higher energy state. When the electron falls back down to its original energy level, it spits out a photon (i.e. light wave). The light that gets reflected into the eye of the observer is the color that the object appears to be. The exact electron configuration determines the wavelength of light reflected, and so determines what color an object is.
Electrons also explain intermolecular interactions between substances, in the form of polarity and Van der Waals forces. Polar molecules have partial charges due to the uneven distribution of electrons. The polar interactions between molecules affect their freezing point, melting point, boiling point, specific heat capacity, and thermodynamic rate of absorption.
Lastly, electron also determine molecular geometry. In covalently bonded compounds, electron will spread out as to minimize the electric potential between themselves. The result is that if one knows how electrons are arranged in a compound, one can predict what geometric shape a molecule of that compound has. The branch of chemistry focused on predicting geometric shape from electron arrangement is called VESPR theory.
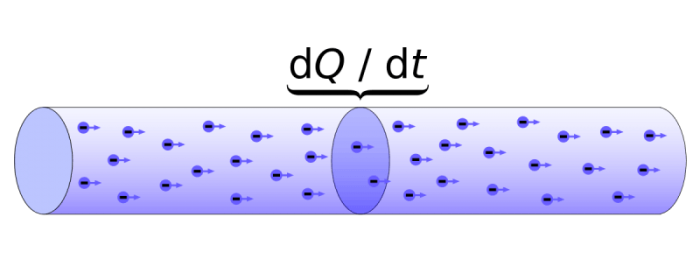
Electric current is the flow of electrons through a body. Credit: “Electric charge and electric current” via WikiCommons CC0 1.0
Electrons are also the main body responsible for the phenomena of electricity. An electrical current consists of the movement of negatively charged electrons through a substance. The movement of charged particles produces work, so the energy from electricity can be harnessed to accomplish physical tasks.
History Of The Electron
Humans have known about electrical phenomena since at least the Ancient Greeks. Pliny the Elder demonstrated that a heated piece of amber would attract small pieces of sawdust and repel shards of iron. It is only within the last 300 or so years that we have developed a sophisticated theoretical understanding of the causes of electrical forces.
In the 1700s, experiments with static electricity led scientists to think that electric forces were caused by two types of opposing fluids called vitreous and resinous, that could be separated by friction and would attract each other. It was around this time that Benjamin Franklin suggested that the two fluids were actually a single fluid with positive excess (+) or a negative deficit (−), thus giving our modern day notations of positive and negative charge.
In the 1800s, scientists began to accept a picture of the atom as composed out of subatomic particles with unit electric charges. In 1874, Irish physicist George Stoney theorized that there existed some particle with a fundamental unit of negative electrical charge, a theoretical particle that he first dubbed the “electrolion” and later “electron.” At the time, Stoney did not have any definitive evidence for the existence of such a particle but postulated it based on how electricity is conducted between objects.
The electron as we understand it nowadays was discovered and identified by British physicist J.J Thompson. In 1897, Thompson demonstrated that cathode rays were composed of negatively charged particles that had very large charge relative to their mass. Thompson originally wanted to call the particles “corpuscles,” but other scientists preferred the name “electron,” which Stoney had suggested 9 years earlier. In 1911, Robert Millikan published his results of his famous oil drop experiment that accurately measured the exact charge of a single electron.
In 1913, Danish physicist Niels Bohr theorized that electrons exist around atoms in definite energy states, giving rise to the first quantum mechanical picture of the electron. In 1924, Louis de Broglie published his doctoral thesis, in which he hypothesized that all particles, electrons included, had a dual wave-particle nature. This realization led Erwin Schrödinger in 1926 to develop the mathematical notion of the wave function, a mathematical function that describes the wave-like behavior of the electron. Further work on Schrödinger’s wave function equation led to the discovery of the antiparticle of the electron, the positron. The current understanding of the electron is encapsulated in modern quantum electrodynamics, a field pioneered by the physicist Richard Feynman.