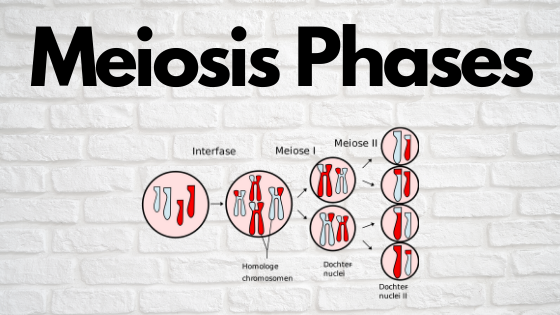
Meiosis refers to the process by which a single diploid cell divides into 4 haploid cells, each genetically distinct from the parent cell. Meiosis is an important part of sexual reproduction in eukaryotes as it provides the raw genetic materials that combine to create a diploid zygote. Unlike mitosis, which is the normal process of cell division, the haploid cells created by meiosis, called gametes, have half the normal number of chromosomes. In humans, a normal body cell has 46 chromosomes (2 sets of 23), so a human gamete only has 23 chromosomes.
“Life is the division of human cells, a process which begins at conception.” — Dick Gephardt
Meiosis itself is divided into 2 major phases, meiosis I and meiosis II, each divided into 4 sub-phases, prophase, metaphase, anaphase, and telophase. Meiosis is similar to mitosis in that both involve cellular division, but is different in two fundamental ways: Mitosis creates two genetically identical cells each with a full set of chromosomes while meiosis shuffle the genes in the chromosomes to create haploid cells genetically distinct from the parent cell and produces cells with half the normal number of chromosomes. In humans, the haploid gametes are sperm and eggs. Sperm and eggs contain half of a complete human genetic code and will fuse their chromosomes to create a diploid zygote.
Meiosis II occurs after meiosis I. During meiosis I, a normal cell splits and results in the formation of two daughter nuclei. During meiosis II, the two daughter cells are split further into 4 distinct haploid cells, each with a single set of 23 chromosomes. Meiosis II is mechanically similar to meiosis I, but has a fundamentally different outcome; the formation of 4 distinct haploid gametes with a unique combination of DNA. Without meiosis II, gametes would not be produced and sexual reproduction as we know it would be impossible.
Overview Of Meiosis I & II
Interphase
Interphase is just the name for the normal life cycle of a cell. During interphase, a cell does all of its normal functions: processing nutrients, construct proteins based on DNA and producing ATP. As interphase draws to a close, and it is time for a cell to reproduce, the cell will duplicate its chromosomes, so that the cell will have 2 full sets of identical sister chromatids.
Meiosis I
Prophase I
Prophase I is the next stage of meiosis I. During prophase I, homologous chromosomes, one that has been inherited from the father and the other from the mother, meet up and exchange bits of DNA. This chromosome crossover is the main mechanism that gives rise to genetic variation among sexually reproducing species. Prophase I is itself divided into 5 smaller sub-phases, each named after the appearance of the chromosomes during that phase.
Metaphase I
During metaphase I, the newly combined homologous chromosomes are moved to the equatorial plane of the cell by the microtubule spindle apparatus. The distribution of homologous chromosomes and sister chromatids along the equatorial plane during cell division is the physical basis for the combinatorial allele distributions described by Mendel’s mathematical laws of inheritance.
“I like nonsense; it wakes up the brain cells.” — Dr. Seuss
Anaphase I
During anaphase I, the homologous chromosomes are pulled apart and are split into two distinct daughter nuclei, each containing one of the homologous chromosomes and attached sister chromatids. During this phase, the cell membrane elongated preparing for its physical division.
Telophase I
Telophase I marks the endpoint of the first meiotic division. in telophase I, the pulled apart homologous chromosomes reach the poles of the cell. The meiotic spindle dissolves and a distinct nuclear membrane surrounds each haploid set of chromosomes, creating two distinct haploid cells in a process called cytokinesis. Some cells do not engage in cytokinesis and instead jump straight to meiosis II
Meiosis II
Meiosis II is a mechanically similar process to meiosis I but the final products are fundamentally different. Meiosis II involves the separation of sister chromatids in the two daughter nuclei produced during meiosis I into 4 distinct haploid cells, each with one set of 23 chromosomes. Meiosis II is also divided into its own prophase, metaphase, anaphase, and telophase. Unlike meiosis I, meiosis II does not begin with the duplication of any chromosomes.
Prophase II
Prophase II is mechanically similar to prophase I. During prophase II the nuclear envelope dissolves and a new microtubule spindle attaches itself to and begins to pull the chromosomes away from the poles of the cell.
Metaphase II
During metaphase II, just like in metaphase I, the chromosomes captured by the meiotic spindle are taken from the poles of the cell and lined up along the metaphase plate, the axis along which each daughter nuclei will split. Once again, the exact spatial distribution along the metaphase plate is the physical basis for the observed combinatorial patterns observed in allele distributions according to Mendel’s laws of inheritance.
Anaphase II
Anaphase II marks the point where the captures sister chromatids are pulled apart by the meiotic spindle and moved towards the poles of their respective cells. and the nuclear membrane elongates to prepare for separation.
Telophase II
Telophase II, like telophase I, is the last stage of the meiotic phase. In telophase II, the nuclear membrane envelops each of the newly separated sister chromatids, creating 4 distinct haploid cells, each with a distinct genetic code.
Products of Meiosis II
The final products of meiosis II (gametes) are the biological entities that make sexual reproduction as we know it possible. In other words, without a process like meiosis II, sexually reproducing species would not be able to create genetically distinct offspring. Gametes are the cells that combine, each providing a portion of the human genetic code to create a new zygote diploid that will eventually develop into a genetically distinct human being.
Although all the products of meiosis II are gametes, they are not genetically identical. Each gamete created has a unique sample of DNA in their single chromatid. The reason for the genetic uniqueness of each gamete is because of the chromosomal crossing over that takes place between homologous chromosomes during pro- and metaphase I. The overlaying of homologous chromosome pairs is capable of creating over 8 million distinct genetic combinations. Combined with the random orientation of homologous pairs during meiosis I, a chromosomal crossover is capable of creating virtually infinite novel genetic combinations.
The presence of these new combinations of chromosomal DNA is what gives rise to genetic variation among species. Each gamete from meiosis contains a chromosome that is genetically distinct from each other and the parent cell. Two gametes (sperm and egg) will combine, creating a completely new set of DNA that has a different combination of alleles. This process is extremely important, as it allows living organisms to have reproduction with variation, a necessary component of evolution. If reproduction did not produce offspring with variations in genotype, then natural selection could not select for beneficial traits.
Errors in the meiotic division are responsible for the majority of miscarriages during pregnancy and development disabilities. Most of these developmental disabilities are the result of an abnormal number of chromosomes, such as trisomy 21 (Down syndrome) or Turner syndrome (missing X chromosome).
Differences Between Mitosis and Meiosis
Mitosis and meiosis are mechanically similar cellular processes but result in fundamentally different sets of products. Most importantly, mitosis produces diploid cells, cells that have a full set of chromosomes for the organisms. Meiosis, on the other hand, produces haploid cells, cells that contain half the number of chromosomes than a normal cell. In humans, a typical diploid cell has 46 chromosomes (2 pairs of 23), so a typical haploid cell has half of that number, 23.
“There are something like 18 billion cells in the brain alone. There are no two brains alike; there are no two hands alike; there are no two human beings alike. You can take your instructions and your guidance from others, but you must find your own path.” — Joseph Campbell
All somatic cells in the body such as skin cells, liver cells, heart cells, and muscle cells undergo mitosis as a form of reproduction. During mitosis, there is no homologous chromosome crossover, so cells formed via mitosis are genetically identical and contain 2 pairs of identical chromosomes. A single mitotic event will create 2 genetically identical cells. Mitosis is one of the main mechanisms of cellular repair and is how the body replenishes and replaces cells that have died or been removed.
In contrast, only a select special group of cells undergoes meiosis. In eukaryotes, those cells are normally found in the gonads; whichever organs are responsible for sexual reproduction. In mammals, meiosis occurs in cells known as oocytes (in females) and in the seminiferous tubules of the testicles in males. Meiosis, as opposed to mitosis, creates 4 haploid cells, each with a distinct genetic profile. The genetic diversity that is present in the products of meiosis is due to the chromosomal crossover of the homologous chromosomes of the parent cell.
In summary, meiosis is a specialized type of cellular division that results in the production of gametes, haploid cells that contain half the normal numbers of chromosomes. One complete turn of the meiotic cycle produces 4 distinct haploid cells from a single diploid cell. Meiosis is important because it creates the raw biological materials that make sexual reproduction possible and provides genetic variation to a population. Chromosomal crossover of homologous chromosomes produces new chromatids with a unique pattern of DNA. Without meiosis, offspring of eukaryotes would be more or less genetically identical.