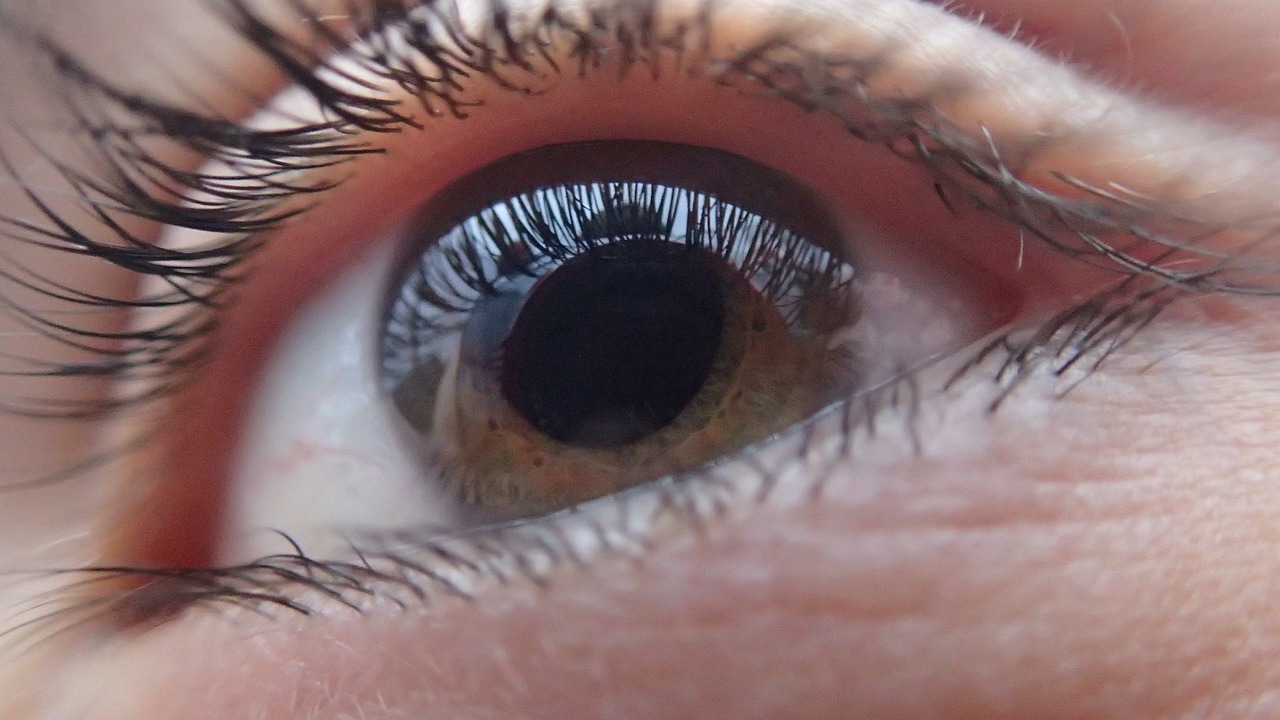
Most of our interactions with the external world are mediated by our visual senses. Approximately 20% of the human neocortex is devoted to the processing of visual information. This is one of the main reasons for the vast and ever-expanding research on visual perception which is dominating the medical and neuroscientific field.
More importantly, from the little annoyance of myopia and presbyopia to the serious impairment of retinal damage or strokes, a large portion of the world’s population experiences suboptimal vision calling for effective solutions to restore visual pathologies.
The majority of current interventions tend to focus on improving the eye’s optics by the use of lenses or invasive refractive surgery. However, recent psychological and neuroscientific advances demonstrated that impaired vision can be similarly improved by simply training the brain to respond more efficiently to the (suboptimal) visual stimulation that reaches the photoreceptors on the retina. This practice, called Perceptual Learning (PL), refers to neural plasticity-induced changes in perceptual abilities due to practice or repetition of a task.
Although the first studies on PL date back to the XIX century (Volkman, 1858; Tawney, 1897), only recently this technique has found its way into clinical settings (Lu, Lin, and Dosher, 2016; Maniglia, Cottereau, Soler, Trotter, 2016). This relatively late application of PL outside of the laboratory might be due to the lack of generalization of the learned material initially found in these studies. While the majority of participants in showed improvements in highly specific parameters learned during the training (retinal position, spatial frequency, and orientation; Sagi, 2011), they were unable to transfer the learned abilities to more general visual functions. Such narrow specificity of improved visual function would be impractical for establishing a rehabilitative technique, given the wide variety of visual stimulations that characterize our daily experience of the natural world.
Despite this potential setback, recent studies still provided evidence of successful PL interventions for visual pathologies such as amblyopia, presbyopia, and myopia. Using low-level visual tasks (e.g., contrast detection; Polat, 2009), PL could compensate for optical defects, presumably by promoting functional neural plasticity. However, in case of visual disorders resulting in irreversible loss of central vision and/or severe difficulties in fine detail vision (Macular Degeneration, Stargardt’s syndrome, Retinitis Pigmentosa), neural compensation for optical aberrations might not be sufficient. Indeed, these conditions might require large-scale cortical reorganization which cannot be achieved by classic PL paradigms, as here the involvement of higher-level visual functions (e.g., motion detection, visual search, etc.) and likely a refocusing of cognitive control mechanisms towards the periphery of the visual field is needed.
Recently, a new type of brain stimulation called transcranial random noise stimulation (tRNS), has been used in combination with PL of low-level visual functions. Using a wide range of frequencies, tRNS delivers weak electric currents to specific cortical regions directly through the scalp. Some studies showed improved visual functions upon employing tRNS in healthy individuals, others successfully used this technique in clinical populations to treat mild visual pathologies such as myopia and amblyopia (Campana et al. 2014, Camilleri et al. 2014). Results from these studies showed that the tRNS stimulated group learned classic PL protocols in fewer training sessions than a non-stimulated group. This constitutes an advantage in clinical settings, as shorter training durations would increase the feasibility of interventions and compliance of patients.
While the exact underlying mechanisms are still being investigated, one hypothesis is that tRNS might keep the stimulated cortical region in a state of higher excitability, thus rendering the affected neurons more responsive to visual stimuli. The combination of classic PL paradigms and tRNS seems to hold the promise for learning effects exceeding the ones previously found with similar techniques and might help patients with central vision loss to overcome their disability. However, no previous study investigated the effect of electric brain stimulation and peripheral, higher-level visual functions. To test the possibility of combining PL and tRNS to effectively improve higher-level peripheral vision, we trained two groups of healthy individuals on a typical peripheral vision task — visual crowding — over the course of four daily sessions. Crowding refers to the difficulty of identifying targets (usually letters) when they are presented in the peripheral field and are flanked on both sides by similar stimuli (non-target letters).
For example, in Figure 1, the H on the left is easy to identify, unlike the one on the right which is flanked by other letters:
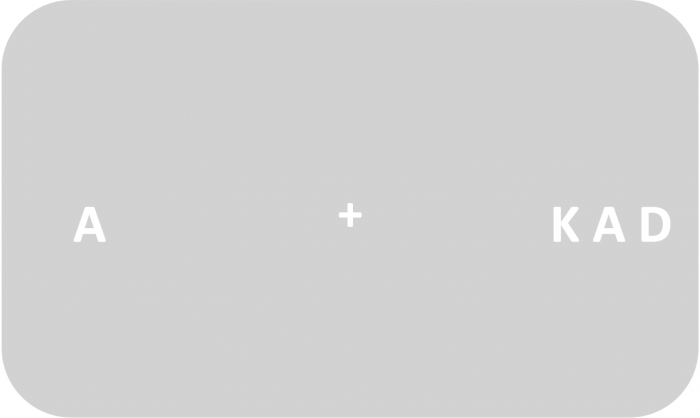
Figure 1: Example of crowded (right) and uncrowded (left) targets: By keeping fixation on the central dot, it is easier to identify the single letter H on the left than the flanked one on the right. Figure courtesy Marcello Maniglia.
Crowding represents one of the main causes of slow reading speed in peripheral vision and its range of effect, called critical space, increases with eccentricity (i.e. distance from the center of the screen to the periphery). This means that the more peripheral the target is placed, the larger the distance between the target and the flankers has to be for them not negatively affecting target identification. Systematic training on crowding usually results in a reduction of critical space. Since impaired reading capability is one of the most common complaints of people with central vision loss, this task represents an ideal probe for the development of effective compensatory interventions.
To test for generalization and a potential transfer of learning, we used similar paradigms, including different stimulus orientations (vertical crowding), different eccentricities, different tasks (uncrowded visual acuity, see Figure 1 left), as well as combinations of those and tested participants before and after training. After four daily sessions of 30 minutes, in which participants were asked to identify the central letter in a triplet of letters (e.g., the H in Figure 1, right) with varying flanker separations, the group who received tRNS was able to identify targets with higher accuracy than the group who did not receive stimulation.
Interestingly, here we observed improvements for different orientations, eccentricities, and tasks, speaking for a transfer of learning.
These results open the possibility of using tRNS to boost PL in peripheral vision, potentially offering an effective solution to patients affected by central vision loss forced to use eccentric vision, or to amblyopic patients, who experience crowding even in foveal vision. While more research is needed to understand the underlying neural dynamics affected by electric brain stimulation, current results employing this technique are encouraging regarding the restoration of visual perception by simple perceptual learning mechanisms.
These findings are described in the article entitled tRNS boosts perceptual learning in peripheral vision, recently published in the journal Neuropsychologia.
Citation:
- G Contemori, Y Trotter, BR Cottereau, M Maniglia (2019). tRNS boosts perceptual learning in peripheral vision. Neuropsychologia(125), 129-136