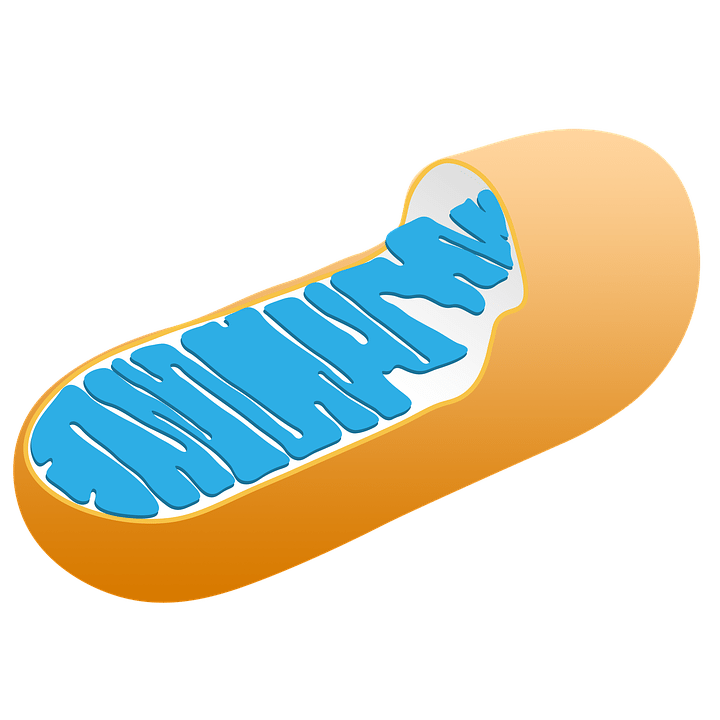
Running the human body takes a fair amount of energy. On average, the human body uses 97.2 joules of energy per second, which comes out to about 8,400,000 joules a day. Where does all this energy come from? Most of the energy in the body comes in the form of ATP, a complex chemical made out a nitrogenous base (adenine) and a 3-phosphate group. Energy derived from ATP is used to drive virtually every process in the body: muscle contraction, digestion, synthesis, growth, etc. Due to its ubiquity in intercellular energy transfer, ATP has been nicknamed the “energy currency of the cell.”
Where does this ATP come from though? The vast majority of ATP production takes place inside the cell. Mitochondria (singular mitochondrion) are cellular organelles whose primary function is to synthesize ATP. The mitochondria are the main site of cellular respiration, the process by which the human body extracts chemical energy out of the food we eat. During cellular respiration, the energy contained in food is converted into the form of ATP, which is then used to do things in the body. If one imagines the body as a city, the mitochondria would be the generators and power plants that give the town its power. Thus, mitochondria are sometimes called the “powerhouse” of the cell.
Mitochondria are found in the cells of most eukaryotic organisms. To date, only one eukaryote is known to lack mitochondria entirely (Monocercomonoides). The exact number of mitochondria in eukaryotic cells can differ depending on the tissue and cell type. Some cells have an abundance of mitochondria (liver cells: ~2,000 ea.) and others might have none (red blood cells). Plant cells typically have fewer mitochondria than other eukaryotes, but they have special organelles called chloroplasts that produce energy. Prokaryotes, on the other hand, lack mitochondria and other organelles.
Mitochondria Structure
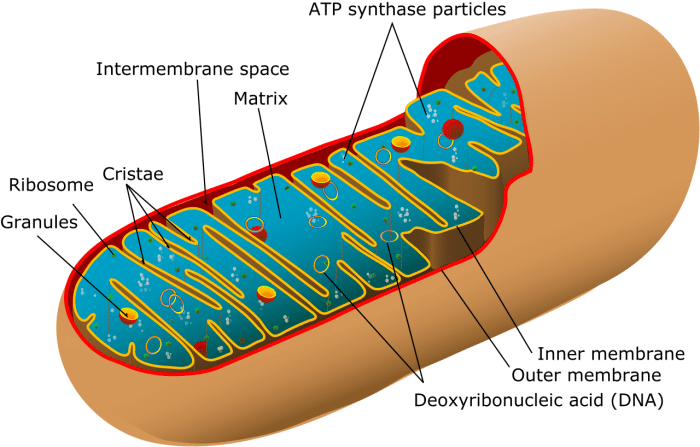
Credit: WikiCommons CC0 1.0
Mitochondria are small organelles (0.75 – 3 μm long) that consist of two phospholipid membranes, the inner and outer membrane. The structure of the inner and outer membrane divides the interior into 5 distinct parts:
- outer membrane
- intermembrane space (between outer an inner membranes)
- inner membrane
- cristae (compartments formed by folds of inner membrane
- matrix space (space inside inner membrane)
Outer membrane: The outer membrane encloses the entire organelle and separates it from the intracellular fluid. The structure of the outer membrane is very similar to the structure of the membrane of the entire cell, composed of two layers of units with hydrophobic lipid heads and hydrophilic phosphate tails. The outer membrane functions to regulate the flow of materials and proteins in and out of the mitochondria. Proteins embedded in the membrane called porins assist with the transport of materials. The outer membrane also contains enzymes that assist with a diverse array of reactions in the cell.
Intermembrane space: Between the outer and inner membrane lies the intermembrane space. Because the outer membrane is permeable to small particles, the fluid composition inside the intermembrane space is similar to that of outside the mitochondria. The intermembrane space also contains proteins transported across the outer membrane.
Inner membrane: Compared to the outer membrane, the inner membrane is composed of many more proteins. The inner membrane contains the proteins that are the site of oxidative phosphorylation, the final step of cellular respiration. The inner membrane is different from the outer membrane because it contains cardiolipin, a 4-fatty acid phospholipid as opposed to the outer membranes 2-fatty acid phospholipids. The extra fatty acids make the inner membrane more impermeable than the outer membrane.
Cristae: The inner membrane contains numerous folds and pockets called cristae. The dense folded shape of the inner membrane into the cristae serves to increase the surface area of the inner membrane which allows for greater ATP production. The exact number of cristae varies depending on how much ATP the cell needs to produce.
Matrix space: The matrix space takes up the most interior part of the mitochondrion and contains 2/3 of the total proteins. The matrix space has a highly concentrated mixture of enzymes, ribosomes, tRNA, and other chemicals. Most of the enzymes function to oxidize pyruvate and fatty acids during the Krebs cycle. The matrix space also contains mitochondrial DNA. Mitochondrial DNA is distinct from the DNA found in the nucleus of the cell.
Although they are normally depicted as small, bean-shaped pods, the actual physical structure of mitochondria can differ greatly depending on the kind of tissue and cell type. Some take on smaller rounder shapes and others look like intricate spirals or amorphous blobs. Mitochondria form dense and complex networks in cells where they are constantly splitting and joining back together.
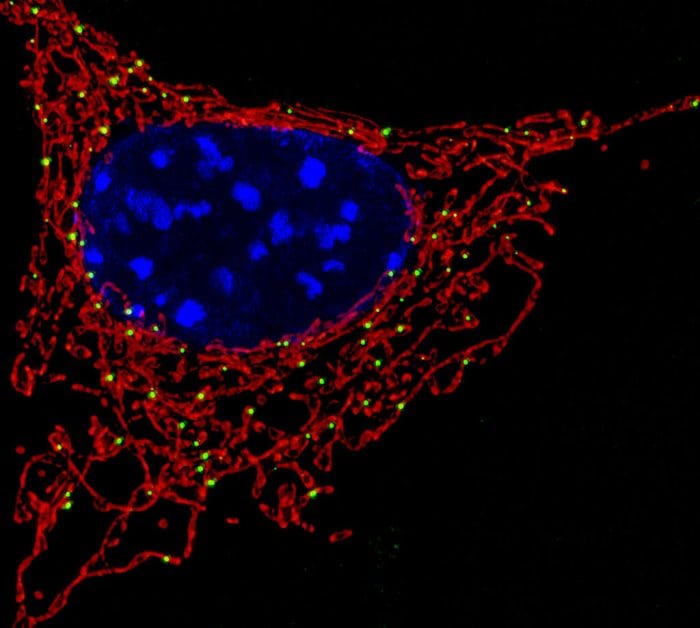
Mitochondria (in red) around the nucleus of a cell (blue). Credit: NICDH via Flickr CC BY 2.0
Mitochondria Function
As stated previously, the main function of mitochondria is to produce ATP. How do they do this? Mitochondria produce ATP as they are the site of a complex series of chemical reactions meant to extract chemical energy from nutrients and convert it into ATP. This process is called cellular respiration. Mitochondria form ATP by oxidizing the products of glycolysis: pyruvate and NADH.
Pyruvate molecules produced during glycolysis are transported across the inner membrane into the matrix space by transport proteins where they are oxidized to make acetyl-CoA and NADH or carboxylated to create oxaloacetate, an important intermediary used in the citric acid cycle. The acetyl-CoA then goes through the citric acid cycle, which in turn produces some ATP and the two electron carriers NADH and FADH2.
The next step is oxidative phosphorylation. The electron carriers deposit their electrons into the proteins in the inner membrane, which creates the electron transport chain, a complex of redox reactions that moves electrons across the inner membrane. The electron transport chain releases energy, which moves protons (H+ ions) across the inner membrane into the intermembrane space. The concentration of protons in the intermembrane space creates a strong electrochemical gradient across the inner membrane. The protons are then shuttled back across the inner membrane by the enzyme ATP synthase. The change in potential energy is used to synthesize ATP from ADP and inorganic phosphates.
The entire process of cellular respiration from glycolysis to oxidative phosphorylation is theoretically capable of producing 36 ATP molecules per single molecule of glucose. The heat of combustion of glucose is 2805 kJ/mol. The heat of combustion of ATP is 30.5 kJ per mole, The efficiency of cellular respiration can be calculated in the following manner:
efficiency = total energy of ATP/total energy of glucose
total energy of ATP = 30.5 kJ/mol x 36 (36 ATP molecules per molecule of glucose) = 1098 kJ/mol
total energy of glucose = 2805 kJ/mol
1098kJ/2805kJ ≈ 39%
Cellular respiration has a theoretical maximum thermodynamic efficiency of about 39%, meaning that over half the energy is lost as heat. In real-world circumstances, respiration is about 34-35% efficient due to inefficiencies in redox reactions and membrane slippage of the electron transport train. In comparison, a standard internal combustion engine operates at about 25-30% thermal efficiency, meaning that mitochondria are a bit more energy efficient than your car.
Mitochondrial DNA
Mitochondria are unique in that they have their own genome that is distinct from that found in the DNA of the cell nucleus. Mitochondrial DNA (mDNA) is stored in a single circular chromosome that resides in the matrix space. Mitochondria have their own mechanisms for DNA replication and transcription.
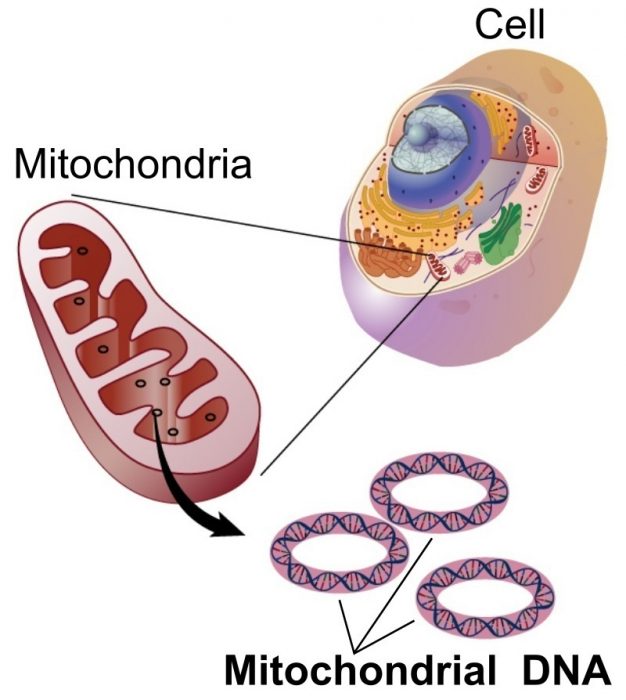
Mitochondrial DNA is distinct from nuclear DNA. Credit: WikiCommons CC0 1.0
In humans, mDNA is almost always passed down from the mother. One reason for this is that human egg cells contain about 1000x more mitochondria that sperm cells. Also, sperm cells contain mitochondria in their flagellum, which can be destroyed during fertilization. In rare cases, a zygote will inherit sets of mDNA from both parents. Compared to nuclear DNA, mDNA is conserved and less likely to undergo mutations.
The fact that mDNA inheritance is virtually always through the mother allows scientists to peer very far back in maternal ancestral lineage. The passage of mitochondrial DNA lets genealogists extrapolate backward to the matrilineal most recent common ancestor (the most recent woman from whom all living humans are descended) who lived about 100,000 – 150,000 years ago. Because all living humans can trace their matrilineal origin to this individual, the matrilineal most recent common ancestor is sometimes called “Mitochondrial Eve” in reference to the biblical creation story.
Where Did Mitochondria Come From?
The origin of mitochondria in eukaryotes is still a debated issue. Currently, the prevailing theory of endosymbiosis states that mitochondria evolved through the symbiosis of different unicellular prokaryotes. This process is called symbiogenesis. According to theories of symbiogenesis, mitochondria and other organelles like chloroplasts were originally free-standing prokaryotic organisms that became incorporated into larger cells. Over time, these engulfed prokaryotes evolved to serve a specialized function in the larger cell.
Several lines of evidence support this theory. Mitochondria, chloroplasts and bacterial cell walls contain the same kind of porins that help shuttle proteins across membranes, both mitochondria and bacterial cell walls contain cardiolipin, mitochondria and chloroplasts only reproduce via binary fission, like prokaryotes, and the circular DNA of mitochondria share many similarities with the circular chromosomes of bacteria.