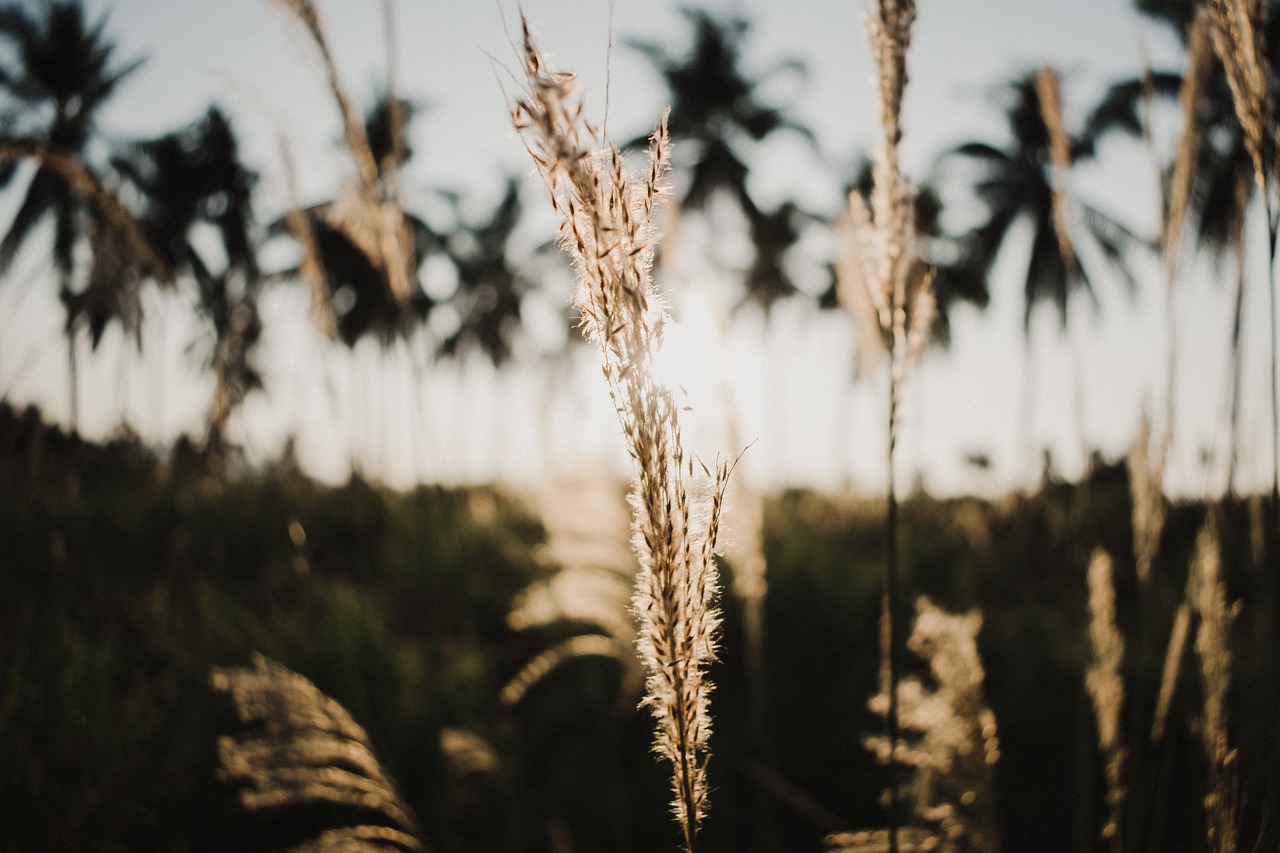
CO2-looping in Biomass Thermochemical Process
Thermochemical processes such as pyrolysis and gasification have been recognized as the most promising fuel processing technique that can utilize biomass for energy recovery. Pyrolysis of biomass can produce biochar, bio-oil, and syngas. Moreover, the by-product of biochar can be fabricated into various carbon materials (e.g., adsorbents, catalysts) for sustainable applications. It was found that CO2, acting as an agent, can participate in each stage (e.g., pyrolysis, gasification) through the integrated thermochemical process, mainly including biomass pyrolysis, biochar gasification, tar cracking/reforming, and gas upgrading (Shen et al., 2017).
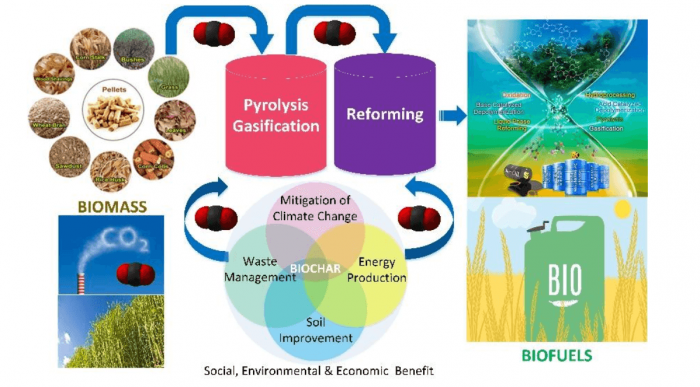
CO2-looping in Biomass Pyrolysis or Gasification (Shen et al., 2017). Image republished with permission from DOI: 10.1039/C7SE00279C.
Biochar By-product Utilization
Biochar by-product with large specific surface area, porous structure, enriched surface functional groups, and minerals make it possible to be utilized as a sorbent to remove pollutants. The conversion of biomass wastes to biochar is a ‘‘win-win’’ strategy for both improving waste management and protecting the environment. Because of its economic and environmental benefits, biochar has become a promising resource for the treatment of pollutants (e.g., heavy metals, organic pollutants) from aqueous solutions.
The properties of biochar mainly depend on biomass types and pyrolysis conditions (e.g., residence time, temperature, heating rate). Conventional carbonization (i.e. slow pyrolysis), fast pyrolysis, and gasification are the main thermochemical processes that are widely used for biochar production. In general, biochar produced at relatively high temperatures (e.g., 600-700 oC) shows highly aromatic with well-organized C layers, but has fewer H and O groups due to dehydration and deoxygenation of the biomass, potentially with lower ion exchange capacities. On the other hand, biochar produced at lower temperatures (e.g., 300-400 oC) has more diversified organic characters (e.g., aliphatic and cellulose type structures) and more C=O and C-H functional groups. The complex and heterogeneous physicochemical composition of biochar can provide an excellent platform for pollutants removal by sorption. In spite of considerable scientific work on the utilize of biochar for environmental uses, extensive attention has recently been concentrated on the modification of biochar with novel structures and surface properties to enhance its remediation efficacy and environmental benefits
Biochar to Activated Carbons
The effectiveness of biochar as an adsorbent has been limited by its low surface area. The production of activated carbons from biomass or biochar has used two activation methods of physical and chemical activation. Physical activation uses oxidizing gases such as air, CO2, and steam, while chemical activation uses chemical activators such as alkali hydroxides (e.g., NaOH, KOH), inorganic acids (e.g., H3PO4, HCl and H2SO4), or metal salts (e.g., ZnCl2). Physical activation is considered to be more environmentally friendly. The carbon precursor is subjected to partial gasification using steam and CO2 as the activating agents. However, physical activation conducted at high temperatures (normally above 900 oC) requires more energy consumption.
Some key points on activated carbons derived from biomass or biochar are concluded: (1) Chemical activation under the inert environment (e.g., N2) is widely used because of its efficient at relatively lower temperatures; (2) Chemical activation contributes to a relatively high specific surface area; (3) KOH is widely used as an activating agent due to its excellent catalytic effect on carbon gasification; and (4) Activation of biochar (i.e. two-step) is considered, since the initial pyrolysis of biomass at a lower temperature causes a high yield of char. Subsequently, the char with poor porosity can be gasified to form many new pores. However, the low-temperature pyrolysis can also yield a high yield of tar. Thus, one-step catalytic pyrolysis with KOH at relatively high temperatures may avoid this problem.
Activated Bio-carbon for Sorption
Researchers from Nanjing University of Information Science and Technology (NUIST) comparatively studied the synthesis of activated biochars from rice husk (an abundant agricultural bio-waste in China) via KOH-catalyzed pyrolysis under the CO2 atmosphere. One-step pyrolysis can produce a higher yield of activated carbon compared with two-step pyrolysis. Pore development in the biochar was significantly improved by the de-ashing process integrated with the KOH activation. Additionally, more pores with deep channels were formed on the surface of activated carbon via one-step pyrolysis due to the K-catalyzed oxidation of carbon structure under the CO2 atmosphere. Therefore, one-step pyrolysis can produce a relatively higher specific surface area (SBET=1836 m2/g) of activated biochar, which showed better performance on the phenol adsorption. As the phenol concentration was 10 mg/L (low), the adsorption capacity of AB1 can reach 75% much higher than that of AB2 (11%). It suggests that the AB1 is more benefit for the removal of phenol with a relatively lower concentration compared with the AB2. However, as the phenol concentration was 50 mg/L (high), the adsorption capacity of AB1 was close to that of AB2.
The potential mechanisms have been proposed by researchers. Biochar obtained at a high temperature is effective in adsorption of organic pollutants by increasing specific surface area, microporosity, and hydrophobicity, while biochar obtained at a low temperature is favored for removing inorganic/polar organic pollutants by the oxygen-containing functional groups, electrostatic attraction, precipitation, and pore-filling. As an organic adsorbate, the phenol molecules are pass into the internal surface via the liquid-film controlled diffusion, so the behavior of phenol adsorption onto activated biochars was mainly controlled via the chemisorption. Biochars normally contain many functional groups with N or O such as -NH2/-OH, C-O, C=O, etc.
Also, the functional groups (e.g., carbonyl, pyrrolic-N groups) on the outer surfaces of activated biochars can attract the phenol molecules onto their internal surfaces via the “π-π dispersion interaction” and “donor-acceptor effect” Hence, the aromatic rings of different phenol molecules are easy to form the π-π stacking interactions. Accordingly, a multi-layer adsorption system is formed. Compared with the Van der Waals force regarded as the primary driving force in the adsorption of activated biochars, the chemical interactions between the functional groups of biochar and phenol molecules are more effective to enhance the adsorption capacity. In addition, the micropores in the internal surface of activated biochar with high specific surface areas host the adsorbed phenol molecules.
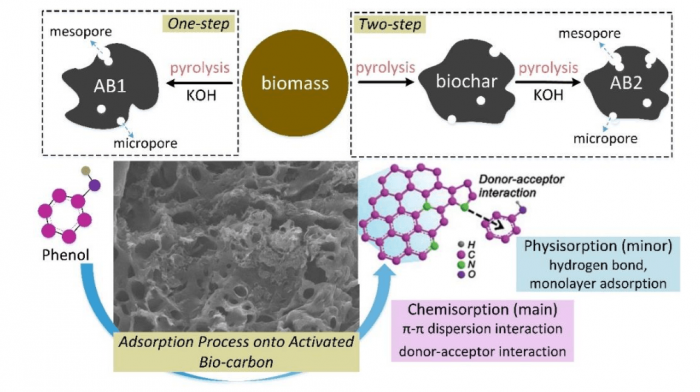
Mechanism of phenol sorption with the activated biochars (AB1: one-step, AB2: two-step) (Shen and Fu, 2018). Image republished with permission from doi.org/10.1016/j.mtener.2018.07.005.
References:
- Yafei Shen, Dachao Ma, Xinlei Ge, CO2-looping in biomass pyrolysis or gasification. Sustainable Energy Fuels 2017, 1, 1700-1729. DOI: 10.1039/C7SE00279C
- Yafei Shen, Yuhong Fu, KOH-activated rice husk char via CO2 pyrolysis for phenol adsorption. Materials Today Energy 2018, 9, 397-405. doi.org/10.1016/j.mtener.2018.07.005