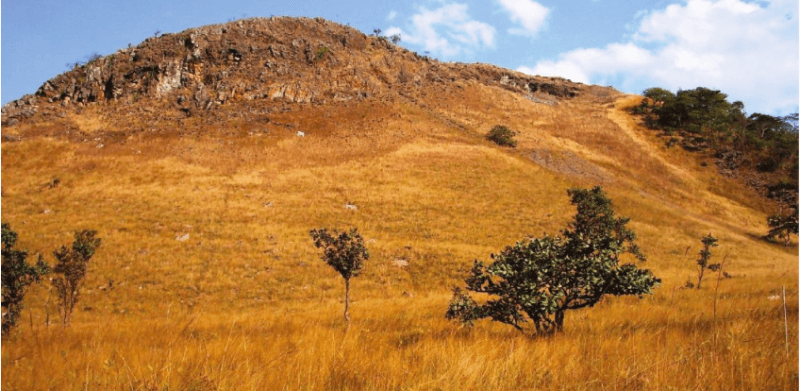
As I explained in my previous article on the environmental impact of the increasing demand for cobalt (Co), mineral resources have been exploited in the Democratic Republic of Congo (DRC) for thousands of years. Such exploitation results in abandoned metalliferous mine wastes with high concentrations of metals. It also results in the contamination of surrounding ecosystems.
To avoid further contamination and health issues, phytostabilization research has been conducted in natural ecosystems of some copper (Cu) hills to evaluate Cu and Co mobility in soil-plant systems.
Metalliferous soils from geological anomalies result from the weathering of rocks that are naturally rich in metals (e.g. Cu and Co in our case — be careful not to consider them heavy metals*). The distribution of Cu and Co in the landscape is driven by various processes, including erosion and vertical transfers in soils. Rock’s evolution into soil depends on two main processes, namely, weathering (physical and chemical alteration) of the parent material and soil formation itself.
The stability of minerals and physico-chemical properties of metals determine their mobility during the weathering process. According to soil conditions (i.e., pH, organic matter content, metal concentrations, redox condition), Cu and Co released from the parent materials are distributed in different soil phases (solid, colloidal, and soluble). Copper and Co mobility also depends on the physicochemical properties of the different phases of soil. Distribution of metals in soil is influenced by pH, total organic carbon, total metal concentration, iron, and manganese oxyhydroxides. These properties and soil constituents can control the mobility and speciation of chemical elements in the soil system. Carbonates, phosphates, iron/manganese oxyhydroxides, and organic carbon are some of the soil constituents that act in the retention and release of metals in soils.
Copper and Co ore deposits are scattered in the south of Katanga province in the DRC. Metalliferous rock fragments are usually located on the tops of hills, and weathering produces various materials rich in Cu and Co that contaminate the surface soil downstream to the slope. Metal transfer in these systems occurs either in particulate, colloidal, or soluble forms. The distribution of vegetation is affected by the concentration of bioavailable Cu and Co forms and chemical factors that influence them.
The diversity of Cu and Co chemical fractions in soil influences plant species diversity by increasing or decreasing Cu and Co bioavailability. The contribution of rock material to surface contamination (natural or anthropogenic) in ecosystems results from contact between a non-contaminated substratum and Cu–Co-rich minerals and subsequent evolution under the physicochemical conditions of the affected substrate. According to their mobility and bioavailability, Cu and Co can be transferred into the soil, water, plants, and, therefore, the food chain.
Nonmetalliferous soils in this part of Katanga are characterized by the woodland known as Miombo. They are exposed to natural contamination sources and anthropogenic contamination sources that are related to mining activities. In this case, contamination may affect the surface layers rich in organic matter (OM) or deeper layers that are poor in OM. The weathering of Cu- and Co-rich minerals, in contact with the soil of these two layers, might release their chemical elements, which will be redistributed in the various phases of the soil. This scenario is typical for the transfer of elements from their parent materials to the soil due to the storage of tailings waste (e.g. waste from mining) but also by what is actually occurring downslope of the outcrops of Cu- and Co-rich rocks in the copper hills ecosystems.
The aim of our study was to go further in the understanding (i) of the reaction between Cu- and Co-rich materials and soils and (ii) of the processes affecting the mobility of Cu and Co in the natural ecosystems. Specific objectives were (i) to assess the potential Cu- and Co-rich rocks’ contribution to soil contamination and (ii) to evaluate the mobility of Cu and Co released into the soil solution according to the properties of soils.
A lysimeter experiment was performed in which a forest soil (Miombo woodland) was artificially spiked with rock fragments from natural Cu and Co hills from Tenke-Fungurume (DRC). The Cu and Co contents of the percolating water were analyzed at repeated intervals, and the impact of rock on the soil properties was evaluated at the end of the experiment.
Five rocks were sampled in one copper hill (Figure 1). In natural conditions, these rock fragments located on the top of the hill are mixed to surface soil horizon along the slope through colluvial processes. The Cu and Co contents in rocks range respectively between 470 mg/kg (siliceous rock) and 140,000 mg/kg (shale) and between 450 mg/kg (dolostone) and 5300 mg/kg (shale).
Rock fragments were mixed with two horizons (hemi-organic A with 2.7% total organic carbon (TOC), and mineral B with 0.3% TOC) of an acid (pH water < 4.5) acrisol under the forest. The mixture was placed in 1 L of lysimeters and left in Lubumbashi ex-situ conditions during the rainy season. Percolating water was collected for six periods afterward, and the Cu and Co contents were analyzed. At the end of the experiment, soil from the lysimeter was removed for pH, TOC, available nutrients and trace elements, CaCl2-extractable Cu, and Co analysis.
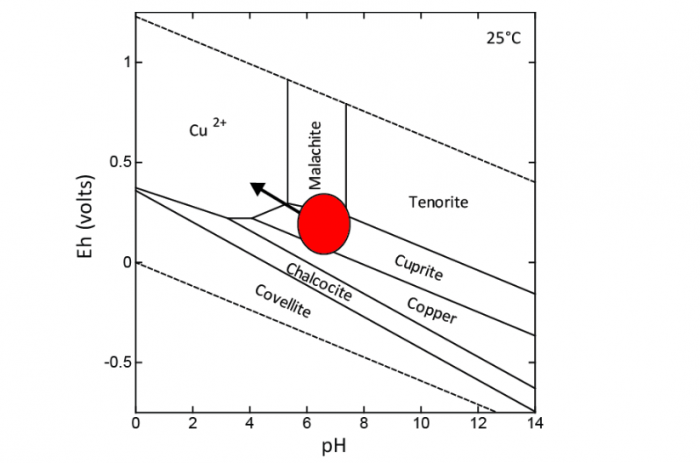
Figure 2. Eh-pH stability diagram of Cu minerals and dissolved species at 25 °C. Image courtesy Olivier Pourret.
Great differences were observed between Cu and Co releases in the percolating solutions according to the nature of the rocks. Released amounts were correlated to unweathered rocks’ concentrations. Differences were also found between the A and B horizons. Soil properties thus influence reaction with the rocks. The main differences between both horizons are organic carbon content, cationic exchange capacity, and nutrient content, which are higher in the A horizon. However, the pH of the A horizon is acidic compared with the B horizon.
Significant correlations were found between extractable Cu and Co with concentrations of their leaching solution. As a consequence, soluble Cu and Co extracted by CaCl2 can be regarded as vertical transfer risk prediction tools of Cu and Co in the soil.
Two additional experiments were conducted under laboratory conditions to characterize the effect of soil properties, mainly pH and total organic content, on metal solubility and sorption on solid phases. Overall, mineralogy controls the supply of elements, pH controls their solubility (Figure 2), and organic matter controls their mobility.
These findings are further described in the article entitled Mobility of copper and cobalt in metalliferous ecosystems: Results of a lysimeter study in the Lubumbashi Region (Democratic Republic of Congo), recently published in the Journal of Geochemical Exploration. This work was conducted by Donato Kaya Muyumba from the University of Liège and Université de Lubumbashi, Olivier Pourret and Jessica Bonhoure from UniLaSalle, Amandine Liénard, Grégory Mahy, and Gilles Colinet from the University of Liège, and Michel Ngongo Luhembwe from the Université de Lubumbashi.
[separator type=”thin”]*Earlier this year, with my colleague Jean-Claude Bollinger, we questioned the use of the term “heavy metal,” and, more recently, I proposed to ban this term from the scientific literature. Why?
In 1980, Nieboer and Richardson proposed replacing this nondescript term with a biologically and chemically significant classification. Moreover, according to the IUPAC, the term “heavy metal” is considered imprecise at best, and meaningless and misleading at worst. The use of this term is strongly discouraged, especially as there is no standardized definition of this term.
The term “heavy metal” is based on categorization by density or molar mass (zinc or copper have relatively low density and molar mass compared to lanthanides and actinides). It is often used as a group name for metals and metalloids (i.e., arsenic) that are associated with contamination and potential toxicity. The “heavy metals” list is not clearly defined and often mixes metals and metalloids without clear definition.
Eventually, the pejorative connotation of “heavy” associated with the toxicity of metal has created a fear in our society. All so-called “heavy metals” and their compounds may have relatively high toxicity. Human exposure to lead by the addition of tetraethyl lead to gasoline or to lead paint is well documented. However, lead-acid batteries do not pose a direct threat to humans but may generate environmentally hazardous waste.
Nonetheless, metals are not always toxic, and some are, in fact, essential: depending on the dosage and exposure levels and the receiving organism/population, a metal may be essential or toxic. Known for its use in the US five-cent coin, nickel is one of the most versatile metals found on Earth. Nickel is essential for life (in some proteins), and its deficiency is accompanied by histological and biochemical changes and reduced iron resorption and may lead to anemia.
To be consistent, researchers should only use well-accepted definitions. In the case of “heavy metal,” this term should be replaced with “metal,” “metalloid,” or “trace metal.” The best way to describe the studied elements is to clearly name them or consider them as a group of elements (metals or metalloids).
References:
- Pourret, O. & Bollinger, J.-C. “Heavy metal” – What to do now: To use or not to use? Sci. Total Environ. 610, 419-420, doi :10.1016/j.scitotenv.2017.08.043 (2018).
- Pourret, O. On the Necessity of Banning the Term “Heavy Metal” from the Scientific Literature. Sustainability 10, 2879, doi:10.3390/su10082879 (2018).