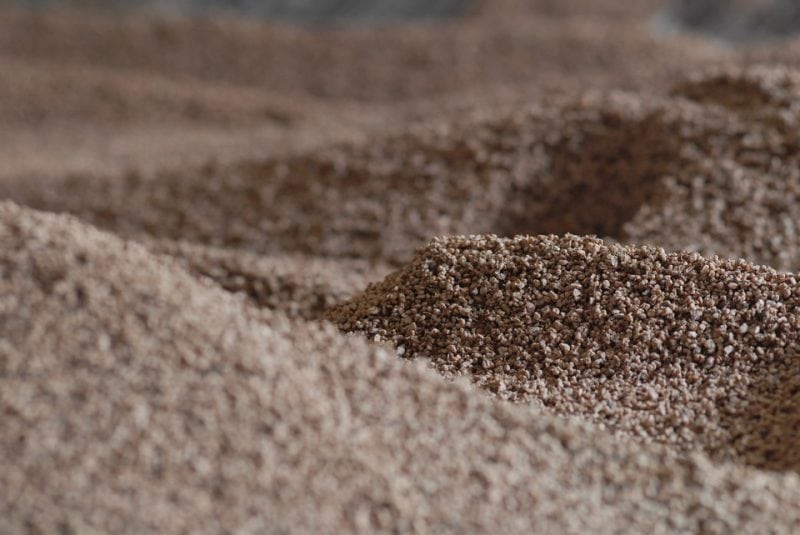
Lignocellulosic biomass has the potential to produce up to 200 billion metric tons worldwide [1] per year. It is acknowledged as one of the most abundant renewable resources on the earth [2]. Lignocellulose is a potential alternative to sustainably replace fossil products such as fuel and chemicals. Lignocellulosic biomass is very diverse and consists complicated carbohydrates [3], but with three basic carbohydrate polymers: (1) hemicellulose, (2) cellulose, and (3) lignin [4].
U.S. Department of Energy supported research at the National Renewable Energy Laboratory (NREL) to identify conversion technologies, which could efficiently utilize biomass to generate base chemicals and subsequently produce fuels, chemicals, and fossil substituted products [5]. The biomass to sugar platform fits the requirement to produce fuels such as ethanol and other building block chemicals.
β-1,4-glycosidic sites connect monomers (glucose) and form cellulose [6]. The OH groups develop intrafiber hydrogen bonds, which are tightly connected and generate water-insoluble crystalline microfibers [7]. Effectively hydrolyzing the crystalline microfibers and producing mainly sugars is recognized as the technical and economic key for the biomass-to-sugar platform [8].
Enzymatic processes are the currently the mainstream of industrialized process to produce bioethanol [9]. The commercialized process only requires low reaction temperature and could contribute to good selectivity [3]. However, the enzymatic process may have a wide range of yields because of the high diversity of feedstock [10]. In addition, the enzymatic method still has a high energy input process [11]. In order to overcome those issues, many types of research are looking for a total solution, for example, a standardization of the process [12], feedstock pretreatments [13-15], and high reaction temperature sustainable enzymes [16, 17].
Concentrated mineral acids (HCl, H2SO4, H3PO4) [18, 19], molten salt hydrates [20], and ionic liquids [21-23] are alternatives to hydrolysis and have been extensively investigated. In 1984, the Purdue Research Foundation patented the hydrolysis of cellulose to glucose using zinc chloride (ZnCl2) [24]. Moreover, the BIOeCON-solvent technology base on molten salt hydrates (ZnCl2) shows economic competitiveness to enzymatic hydrolysis [14]. However, separation hydrolysis sugar from ZnCl2 and recycle ZnCl2 are two main issue to reach economic sustainability [8, 10, 25, 26]. Nevertheless, chemical hydrolysis requires a cost-effective process to deal highly concentrated streams to successfully cosmeticize bioethanol production.
The Industrial Technology Research Institute (ITRI) has developed a nanofiltration system that integrates into the ZnCl2 hydrolysis process as shown in Figure 1, which has advantages of easy scale-up, low capital expenditure (CAPEX), low operating expenses (OPEX), and most importantly, no wastewater. The designed multiple nanofiltration processes can purify sugar from high ZnCl2 stream hydrolysis solution. Moreover, the integrated multiple nanofiltration processes successfully validated that desalinated real bagasse hydrolysis solution is fermentable to produce bioethanol.
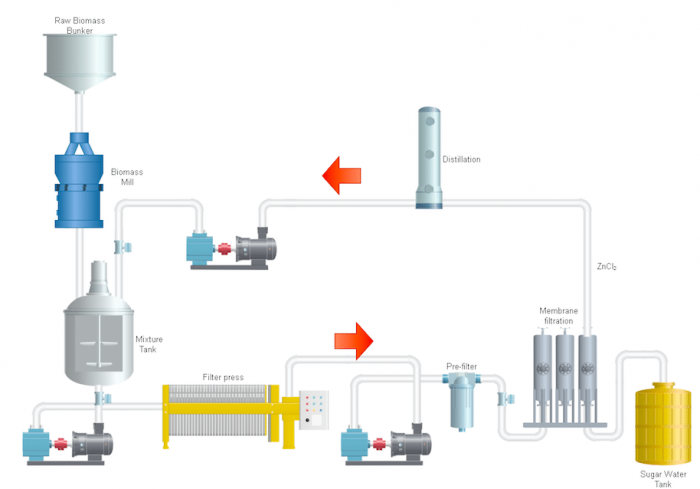
Figure 1 Biomass salt hydrolysis process flow (Credit: Yen-Ting Kuo)
The whole hydrolysis process is simple (Figure 1). The raw biomass is crushed into small pieces and mixed with the ZnCl2+HCl solution, heating up to around 100 oC for a while. Then the filter presses to remove the solid parts: lignin, which could not be hydrolyzed by the acidic salt solution. Now the solution’s main content is glucose, xylose, and ZnCl2. Finally, the following process is applied to the integrated nanofiltration system to remove ZnCl2 and obtain the final product (sugar solution). The ZnCl2 is recycled to the beginning of the process after removing extra water. The distilled water is also recycled to the filtration process and establishes the zero wastewater system.
The desalination of real bagasse hydrolysis solution reaches total 99 wt.% sugar recycled, and ZnCl2 is successfully recycled in permeate. The processed hydrolysis solution achieves an 82% total alcohol yield. The zero wastewater and integrated nanofiltration process can help implement the renewable energy industry in both economic and environmental sustainability. Most importantly, the maturated market make the membranes easily available and scale up.
These findings are described in the article entitled Technical and Economic approach of bioethanol production from nanofiltration of biomass chemical hydrolysis solutions, recently published in the journal Applied Energy. This work was conducted by Yen-Ting Kuo, Ju-Shiou Chen, Tzu-Yueh Yang, and Hou-Peng Wan from the Industrial Technology Research Institute. The corresponding author currently works at AX Group, which is one of the leading companies for sludge and wastewater treatment in Taiwan.
References:
- Ragauskas AJ, Williams CK, Davison BH, Britovsek G, Cairney J, Eckert CA, et al. The path forward for biofuels and biomaterials. Science. 2006;311:484-9.
- Zhao X, Cheng K, Liu D. Organosolv pretreatment of lignocellulosic biomass for enzymatic hydrolysis. Appl Microbiol Biotechnol. 2009;82:815-27.
- Fatih Demirbas M. Biorefineries for biofuel upgrading: A critical review. Applied Energy. 2009;86:S151-S61.
- Yang H, Yan R, Chen H, Zheng C, Lee DH, Liang DT. In-Depth Investigation of Biomass Pyrolysis Based on Three Major Components: Hemicellulose, Cellulose and Lignin. Energy & Fuels. 2006;20:388-93.
- Fernando S, Adhikari S, Chandrapal C, Murali N. Biorefineries: Current Status, Challenges, and Future Direction. Energy & Fuels. 2006;20:1727-37.
- Guerriero G, Hausman J-F, Strauss J, Ertan H, Siddiqui KS. Lignocellulosic biomass: Biosynthesis, degradation, and industrial utilization. Engineering in Life Sciences. 2016;16:1-16.
- Parthasarathi R, Bellesia G, Chundawat SP, Dale BE, Langan P, Gnanakaran S. Insights into hydrogen bonding and stacking interactions in cellulose. J Phys Chem A. 2011;115:14191-202.
- Zhang YH, Ding SY, Mielenz JR, Cui JB, Elander RT, Laser M, et al. Fractionating recalcitrant lignocellulose at modest reaction conditions. Biotechnol Bioeng. 2007;97:214-23.
- Favaro L, Basaglia M, van Zyl WH, Casella S. Using an efficient fermenting yeast enhances ethanol production from unfiltered wheat bran hydrolysates. Applied Energy. 2013;102:170-8.
- Frankó B, Galbe M, Wallberg O. Bioethanol production from forestry residues: A comparative techno-economic analysis. Applied Energy. 2016;184:727-36.
- Budzianowski WM, Postawa K. Total Chain Integration of sustainable biorefinery systems. Applied Energy. 2016;184:1432-46.
- de Carvalho AL, Antunes CH, Freire F. Economic-energy-environment analysis of prospective sugarcane bioethanol production in Brazil. Applied Energy. 2016;181:514-26.
- Domínguez E, Romaní A, Domingues L, Garrote G. Evaluation of strategies for second generation bioethanol production from fast growing biomass Paulownia within a biorefinery scheme. Applied Energy. 2017;187:777-89.
- Akobi C, Yeo H, Hafez H, Nakhla G. Single-stage and two-stage anaerobic digestion of extruded lignocellulosic biomass. Applied Energy. 2016;184:548-59.
- Wirawan F, Cheng C-L, Kao W-C, Lee D-J, Chang J-S. Cellulosic ethanol production performance with SSF and SHF processes using immobilized Zymomonas mobilis. Applied Energy. 2012;100:19-26.
- Tran D-T, Chen C-L, Chang J-S. Continuous biodiesel conversion via enzymatic transesterification catalyzed by immobilized Burkholderia lipase in a packed-bed bioreactor. Applied Energy. 2016;168:340-50.
- Xu Y, Wang D. Integrating starchy substrate into cellulosic ethanol production to boost ethanol titers and yields. Applied Energy. 2017;195:196-203.
- Moe ST, Janga KK, Hertzberg T, Hägg M-B, Øyaas K, Dyrset N. Saccharification of Lignocellulosic Biomass for Biofuel and Biorefinery Applications – A Renaissance for the Concentrated Acid Hydrolysis? Energy Procedia. 2012;20:50-8.
- Orozco A, Ahmad M, Rooney D, Walker G. Dilute Acid Hydrolysis of Cellulose and Cellulosic Bio-Waste Using a Microwave Reactor System. Process Safety and Environmental Protection. 2007;85:446-9.
- <2017 [Industrial & Engineering Chemistry Research] Production of monosugars from lignocellulosic biomass in molten salt hydrates process.pdf>.
- Liu C-Z, Wang F, Stiles AR, Guo C. Ionic liquids for biofuel production: Opportunities and challenges. Applied Energy. 2012;92:406-14.
- Baral NR, Shah A. Techno-economic analysis of cellulose dissolving ionic liquid pretreatment of lignocellulosic biomass for fermentable sugars production. Biofuels, Bioproducts and Biorefining. 2016;10:70-88.
- Vancov T, Alston A-S, Brown T, McIntosh S. Use of ionic liquids in converting lignocellulosic material to biofuels. Renewable Energy. 2012;45:1-6.
- Li F. Chen WL, Ind.; Che-Ming Yang, Clark, N.J. . SELECTIVE HYDROLYSIS OF CELLULOSE TO GLUCOSE WITHOUT DEGRADATION OF GLUCOSE USING ZINC CHLORIDE. . US: Purdue Research Foundation, West Lafayette, Ind.; 1985.
- Choi IS, Lee YG, Khanal SK, Park BJ, Bae H-J. A low-energy, cost-effective approach to fruit and citrus peel waste processing for bioethanol production. Applied Energy. 2015;140:65-74.
- Liu Y, Zhang Y, Xu J, Sun Y, Yuan Z, Xie J. Consolidated bioprocess for bioethanol production with alkali-pretreated sugarcane bagasse. Applied Energy. 2015;157:517-22.