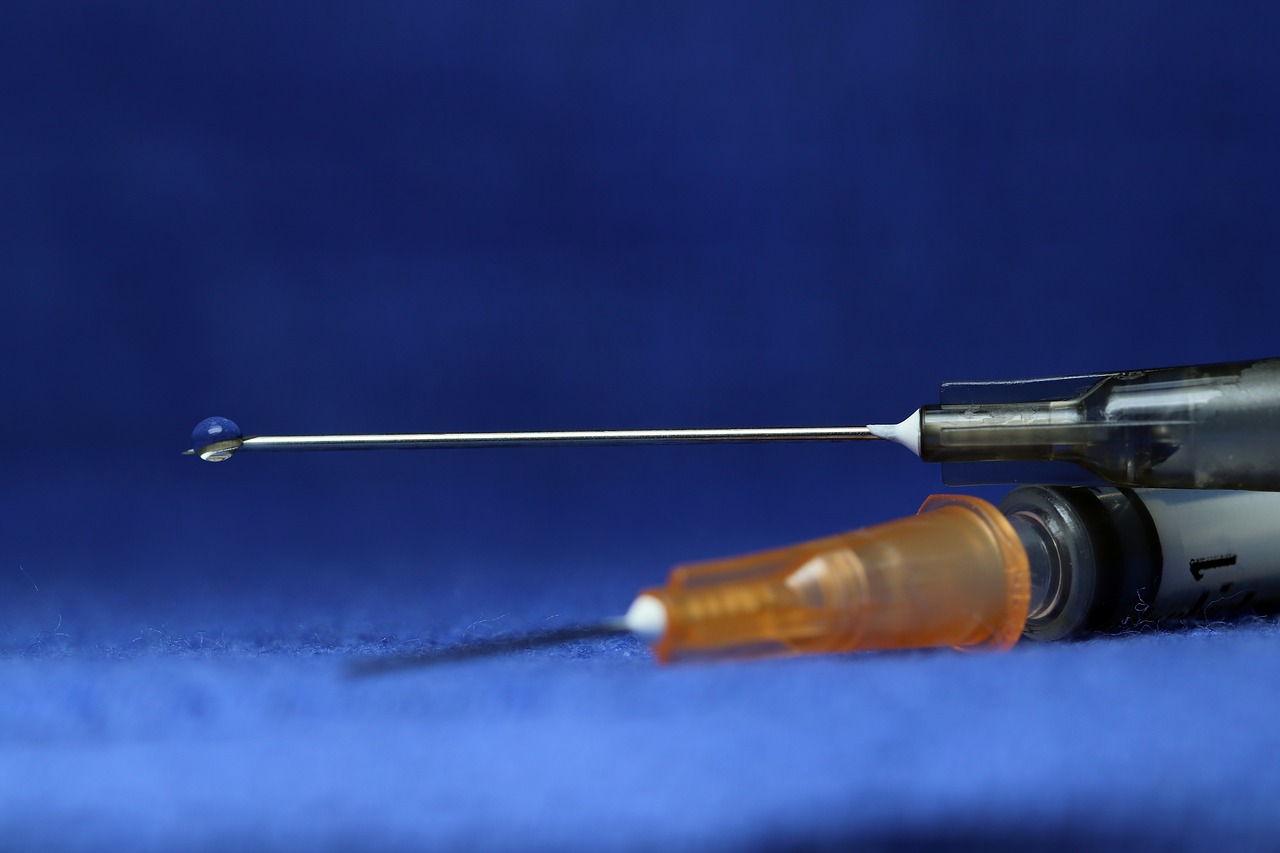
Nanoscience and nanotechnology offer extremely optimistic possibilities to be applied in various disciplines of science and technology. Nobel laureate Richard Feynman laid the foundation for nanotechnology with his famous lecture, “There’s Plenty of Room at the Bottom,” at the California Institute of Technology (CalTech) on December 29, 1959, during the annual meeting of American Physical Society. Prof. Feynman urged the scientific community to “think small,” long before the term nanotechnology was coined. The field of nanotechnology began to reach newer dimensions with the advancement of electron microscopy techniques.
Nanotechnology has emerged as a hot topic of scientific research by the end of the 20th century (1). Nanoparticle (NP) based products such as electronic devices, optical devices, sensors, clothes, sunscreens, food, and medicines have already flourished in our day-to-day life. The Nanotechnology Consumer Product Inventory (CPI) reported that more than 1600 nanotechnology-derived products were on the market in 2015 (2).
Nanotoxicology constitutes a relatively novel branch of toxicology which deals with the toxicity of NPs. Wider applications of NPs simultaneously broaden the risk factors associated with them. Metal oxide NPs comprise a major class of NPs which are produced principally at the industrial level. Well-known examples include zinc, titanium, iron, silver oxide, etc. Justification for the rising popularity of these NPs lies in the versatility in forming various sizes and shapes according to the synthesizing conditions. Their smaller size and high edge surface sites add to their popularity. Metal oxide NPs are widely used in industrial as well as health care applications. There are several products containing metal oxide NPs, such as iron oxide NP-based MRI contrast agents and cosmetics containing zinc and titanium oxides (3).
Human exposure to NPs occurs from the ambient air, consumer goods, manufacturing process, and through intentional administering in the form of drugs and diagnostic modalities. The same physico-chemical properties which give them enormous potential in bio-applications also give rise to their toxicity. NPs may interact with the biological system in unpredictable ways due to its small size, high surface-area-to-volume ratio and quantum confinement. For instance, gold is an inert material, whereas gold NPs are highly reactive (4). Being smaller in size, these NPs may travel throughout the body and access otherwise protected areas like the cell nucleus, blood-brain barrier (BBB), and placenta.
One of the prominent cellular mechanisms of NP cytotoxicity is by means of reactive oxygen species (ROS) generation and resulting oxidative stress. Under normal circumstances, this ROS will be eliminated by antioxidant machinery present in the cell, which could worsen if the cell undergoes an NP invasion. Another possible toxicity mechanism exhibited by NPs includes intracellular dissolution, which could bring about toxic ions in circulation. This sometimes disrupts metal homeostasis, and the ions released may interfere with enzyme activities (5).
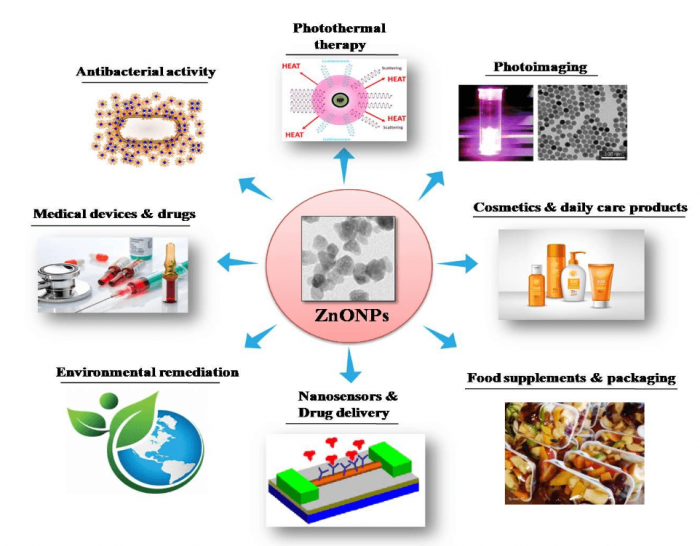
Figure 1. Application potentials of ZnO NPs in various industrial and medical fields. Image courtesy Mohanan PV.
ZnO NPs constitute an extremely hopeful group of NPs which hold tremendous application potentials. Different application fields of ZnO NPs are illustrated in Figure 1. As an NP in high demand, toxicity studies on ZnO NPs have received much attention. Even though plenty of studies have been done on toxicity assessments for ZnO NPs in various cell types in vitro, very few studies have been devoted to their neurotoxic potential. It has been reported earlier that ZnO NPs interact with neurons affecting the voltage-gated channel and synaptic plasticity.
It is also widely accepted that ZnO NPs prefer in vivo olfactory neuronal pathways for gaining entry into the brain where it stimulates inflammatory responses (6). Among the various cell types residing in the brain loci, astrocytes represent the most abundant glial cell community which performs a variety of functions in CNS ranging from axon guidance and synaptic support to control of BBB integrity and metal homeostasis (7). A generalized diagram showing adverse effects of NPs in the brain is illustrated in Figure 2. The present study investigates the neurotoxic potential of ZnO NPs during interaction with primary astrocytes isolated from post-natal 0-2 day old rat pups. This further enhances the magnificence of the work, since it stands closely with the realistic environment in a living system.
ZnO NPs were generated by a wet precipitation method and characterized both physicochemically and biologically via different urbane techniques. Homogenous distribution and colloidal stability of the particles were clearly evident from the results obtained. Astrocytes were isolated from rats using a protocol proposed by Weinstein DE (2001) (8) and were characterized via immunostaining using different cellular markers. Cell viability assays (MTT and NRU assays) confirmed the time and dependent reduction in viability, suggesting metabolic instability. Possible morphological alterations were then evaluated and confirmed by fluorescent and non-fluorescent staining techniques.
In conjunction with viability studies, significant alterations were clearly visible like reduction in cytoplasm, nuclear condensation, cell shrinkage, and actin re-organization. Subsequent investigation of lysosomal stability was evaluated using acridine orange staining and ensured active lysosomal integrity. NPs were capable of generating ROS, which was confirmed using a DCF probe, while it remained inactive in eliciting reactive nitrogen species (RNS) generation.
Apoptotic cell death was found to be originated from significant loss in mitochondrial membrane potential. Nuclear premises of the cell remained unaffected without any nuclear condensation or fragmentation. A comparative study was conducted for the toxic potential of ZnO NPs, ZnCl2, and ZnO bulk form. This further cemented the fact that ZnO NPs are capable of eliciting a nano-specific toxic response irrespective of its dissolution state. Our overall study concludes that ZnO NPs could bring out toxic response while interacting with astrocytes of brain. This adds concern for compromised immunity and key functionalities of the brain during exposure with ZnO NPs.
References:
- Bao G, Mitragotri S and Tong S (2013) Multifunctional nanoparticles for drug delivery and molecular imaging. Annual review of biomedical engineering 15: 253-282.
- Vance ME, Kuiken T, Vejerano EP, McGinnis SP, Hochella Jr MF, Rejeski D and Hull MS (2015) Nanotechnology in the real world: Redeveloping the nanomaterial consumer products inventory. Beilstein journal of nanotechnology 6: 1769-1780.
- Fernández‐García M and Rodriguez JA (2011) Metal oxide nanoparticles. Encyclopedia of Inorganic and Bioinorganic Chemistry.
- Panyala NR, Peña-Méndez EM and Havel J (2009) Gold and nano-gold in medicine: overview, toxicology and perspectives. J Appl Biomed 7: 75-91.
- Soenen SJ, Rivera-Gil P, Montenegro J-M, Parak WJ, De Smedt SC and Braeckmans K (2011) Cellular toxicity of inorganic nanoparticles: common aspects and guidelines for improved nanotoxicity evaluation. Nano Today 6: 446-465.
- Kao Y-Y, Cheng T-J, Yang D-M, Wang C-T, Chiung Y-M and Liu P-S (2012) Demonstration of an olfactory bulb–brain translocation pathway for ZnO nanoparticles in rodent cells in vitro and in vivo. Journal of Molecular Neuroscience 48: 464-471.
- Blackburn D, Sargsyan S, Monk PN and Shaw PJ (2009) Astrocyte function and role in motor neuron disease: a future therapeutic target? Glia 57: 1251-1264.
- Weinstein DE (2001) Isolation and purification of primary rodent astrocytes. Curr Protoc Neurosci, 3.5. 1-3.5. 9.