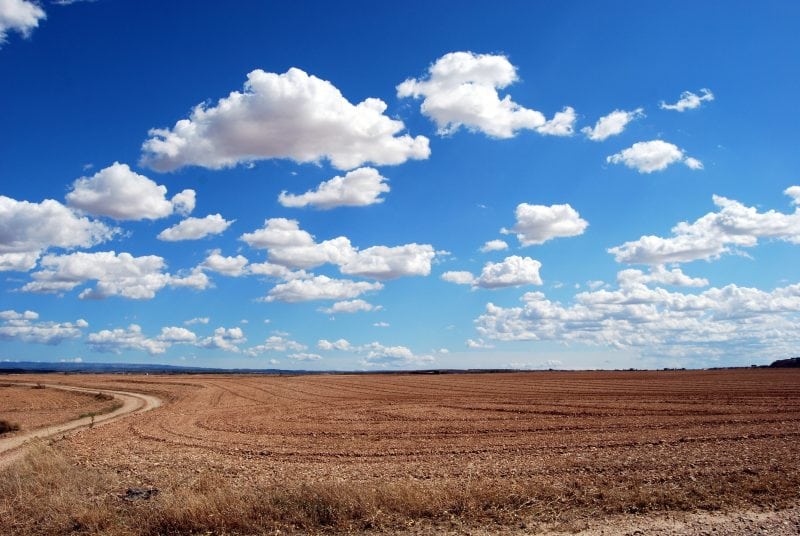
Observing and mathematically describing our environment provides a foundation for developing sustainable future scenarios. These aim to address crucial socio-economic resources such as water, food and energy security, as well as forecasting of severe weather and climate, and strategies for human health and adaptation (e.g., Pielke Sr et al., 2016). Present-day examples include large-scale missions to monitor the earth system such as NASA ECOSTRESS and Oil and Gas industry methane leak detection, among others.
At times, however, existing mathematical descriptions are at odds with the way we actually observe our environment. For example, the first law of thermodynamics, the conservation of energy (and in extension, mass), is often expressed in the form of a Cartesian control volume, or box (Figure 1). This is quite convenient, as we can now describe the exchanges of energy and mass by simply adding or subtracting the transfers across all faces of the box to or from the quantity in the box itself. In this example, surface-atmosphere exchange, also known as “flux,” quantifies how heat and gasses are transferred between soil, plants and the atmosphere.
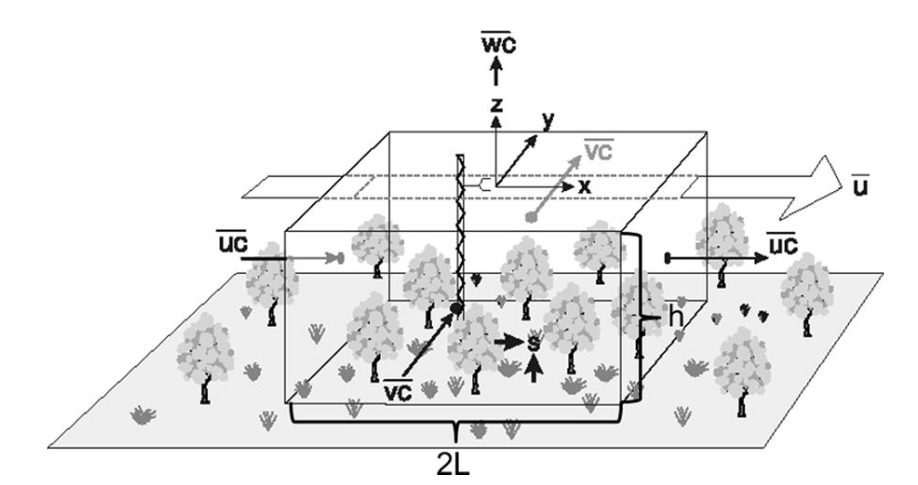
Figure 1†. Conceptual framework of a Cartesian control volume, or box, with length and width 2 L and height h (after Finnigan, 2004). The exchange of heat and gasses between soil, plants and the atmosphere can be quantified by tower observations in the center of the box, in combination with conservation laws. Here, c is a scalar quantity such as temperature, water vapor or carbon dioxide; u, v and w are wind components with respect to the coordinate axes x, y, and z; s is storage, i.e. increase or decrease of the scalar quantity inside the box. The overbars indicate a time average. (Republished with permission from Elsevier)
Eddy-covariance is one of the reference methods that allow us to observe this surface-atmosphere exchange in nature. It uses sensors on towers that gather data on wind, temperature, and gas concentrations. However, instead of a box, these observations actually represent irregular Lagrangian control volumes that change in shape with factors such as wind direction, not unlike smoke plumes (Figure 2). So, essentially, the mathematical description and observation of the surface-atmosphere exchange currently coexist in two languages; we first need a translation so we can then determine reliable mathematical descriptions from the observations for use in our models of the earth system.
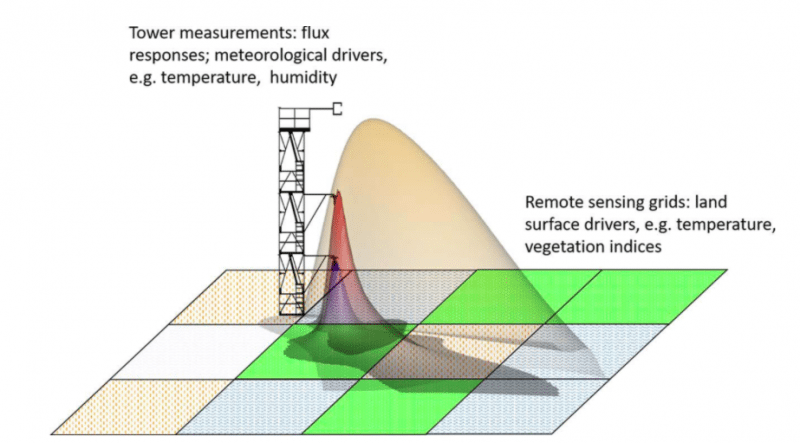
Figure 2†. Eddy-covariance flux measurements from a Lagrangian perspective: instead of a box, we actually observe an upwind control volume that changes its size and shape with factors such as measurement height and wind direction. A novel data-fusion concept links the tower-measured flux response to in-situ meteorological measurements and remotely-sensed surface properties. (Republished with permission from Elsevier)
The environmental response function (ERF) technique in combination with a virtual control volume (VCV) provides a novel approach to accomplish this translation. This is achieved through relating observations of the atmospheric flux “response” once per minute to meteorological “drivers” and remotely-sensed surface properties. The extracted relationships are used to explicitly re-project fluxes in 3-D space and time throughout the VCV, which can then be analyzed in accordance with conservation laws (Figure 3). On a technical level, ERF-VCV accomplishes that by catalyzing process and artificial intelligence concepts: it complements existing mathematical descriptions of better-understood processes such as atmospheric dispersion with observations from towers, aircraft, and satellites about less well-understood phenomena. It then mines the joint information content and yields the most complete solution that is possible from the processes and observations provided.
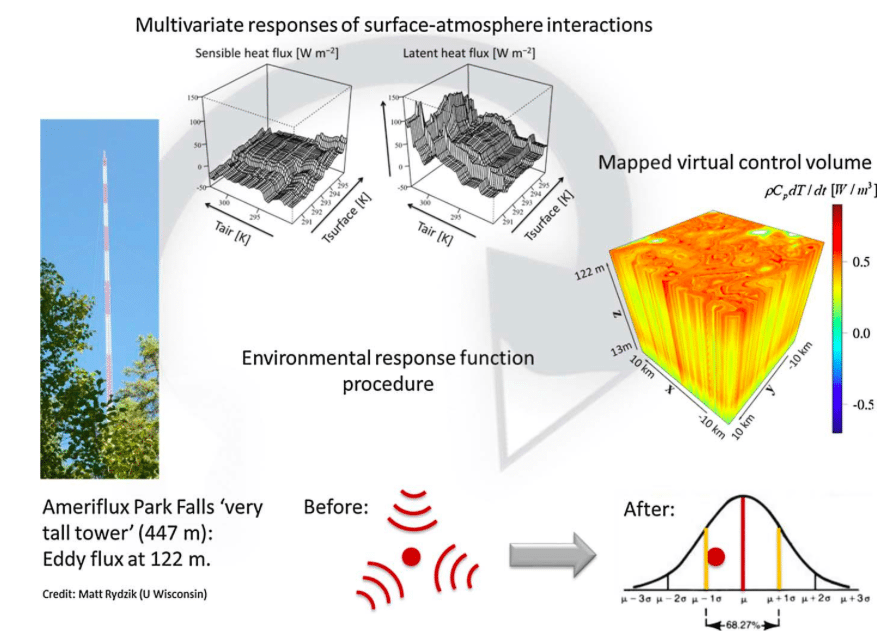
Figure 3†. Essential steps of the ERF-VCV approach, from observation over response function extraction to spatio-temporal projection and resulting spatial probability density function. (Republished with permission from Elsevier)
In result, an answer to a complex question is provided incl. confidence intervals, such as translating actual observations of surface-atmosphere exchange into a mathematical box (Figure 4). The difference between using and omitting the ERF-VCV translation shows promise to address long-standing biases in surface-atmosphere exchange observations on the order of several 10%. This work resulted in the paper “Surface-atmosphere exchange in a box: Making the control volume a suitable representation for in-situ observations” in Agricultural and Forest Meteorology (Metzger, 2018). A follow-up paper “Surface-atmosphere exchange in a box: Space-time resolved storage and net vertical fluxes from tower-based eddy covariance” (Xu et al., 2018) explores the practical application and impact of this novel data-fusion concept.
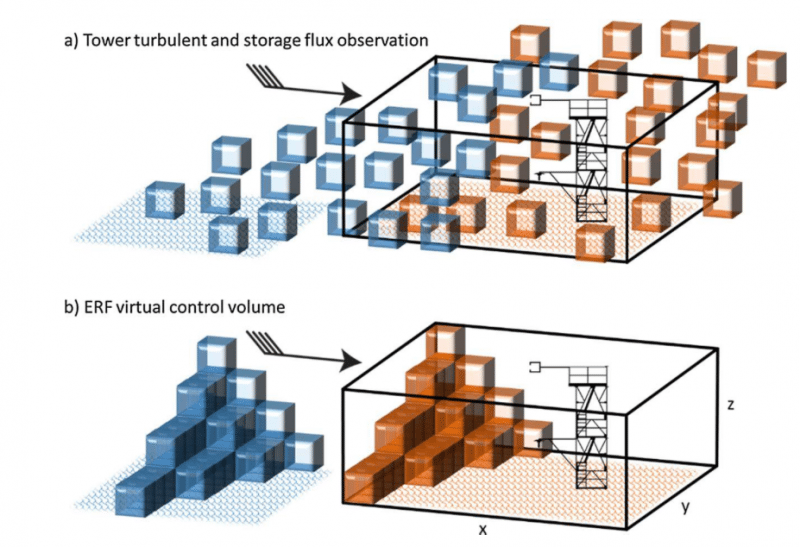
Figure 4†. Eddy-covariance flux measurements in a Cartesian control volume. Top panel: Tower observations in a “leaky” control volume with air parcels from within and beyond the control volume passing by the tower. Bottom panel: ERF-VCV mapping operator independently considers the surface forcing on an air parcel and its atmospheric mediation while holding it horizontally in place. (Republished with permission from Elsevier)
Some recent applications of ERF-VCV modules include the mapping of biogenic and geogenic methane sources in the Arctic (Kohnert et al., 2017; Kohnert et al., 2018), agricultural methane emission inventories (Desjardins et al., 2018), as well as attributing urban emissions of nitrogen oxides and volatile organic compounds (Vaughan et al., 2016; Vaughan et al., 2017).
More generally, ERF-VCV allows bridging substantial information gaps between our different types of observations. For example, tower eddy-covariance measurements provide us detailed information at a local level, but their spatial coverage is very limited – on the order of hectares. On the other hand, with airborne and satellite data one can obtain close to global coverage, but it is delivered in square kilometer increments and intermittent in time. These differences in data characteristics gathered by the various observation systems make it difficult to combine their information in truly synergistic ways. ERF-VCV allows combining in-situ and remote sensing data so that researchers are able to tap into the full complement of available information. Among the prospective applications are methane leak detection for the Oil and Gas industry, and calibration of large-scale earth system monitoring missions such as NASA ECOSTRESS.
Eddy-covariance station networks such as AmeriFlux, NEON, and others are vital for providing the necessary distributed in-situ observations. Each day, this generates millions of data points for each of the 47 terrestrial NEON sites, representing the largest single-provider eddy-covariance tower network globally. Moving forward, an important aspect will be to learn how to use data from various systems and networks more effectively instead of relying on more and more equipment in the field alone. Here, ERF-VCV can live up to its full “machine learning in the Geosciences” potential and can help consolidate the myriads of raw data into products that present the information in meaningful ways.
These findings are described in the article entitled Surface-atmosphere exchange in a box: Making the control volume a suitable representation for in-situ observations, recently published in the journal Agricultural and Forest Meteorology. This work was conducted by Stefan Metzger, Ph.D. from the National Ecological Observatory Network, Battelle, and the University of Wisconsin-Madison.
Acknowledgment
†Reprinted from Agricultural and Forest Meteorology, Volume 255, Stefan Metzger, Surface-atmosphere exchange in a box: Making the control volume a suitable representation for in-situ observations, Pages 68-80, Copyright (2018), with permission from Elsevier.
References
- Desjardins, R. L., Worth, D. E., Pattey, E., VanderZaag, A., Srinivasan, R., Mauder, M., Worthy, D., Sweeney, C., and Metzger, S.: The challenge of reconciling bottom-up agricultural methane emissions inventories with top-down measurements, Agric. For. Meteorol., 248, 48-59, doi:10.1016/j.agrformet.2017.09.003, 2018.
- Finnigan, J. J.: A re-evaluation of long-term flux measurement techniques. Part 2: Coordinate systems, Boundary Layer Meteorol., 113, 1-41, doi:10.1023/B:BOUN.0000037348.64252.45, 2004.
- Kohnert, K., Serafimovich, A., Metzger, S., Hartmann, J., and Sachs, T.: Strong geologic methane emissions from discontinuous terrestrial permafrost in the Mackenzie Delta, Canada, Scientific Reports, 7, 5828, doi:10.1038/s41598-017-05783-2, 2017.
- Kohnert, K., Juhls, B., Muster, S., Antonova, S., Serafimovich, A., Metzger, S., Hartmann, J., and Sachs, T.: Towards understanding the contribution of waterbodies to the methane emissions of a permafrost landscape on a regional scale – a case study from the Mackenzie Delta, Canada, Global Change Biol., accepted author manuscript, doi:10.1111/gcb.14289, 2018.
- Metzger, S.: Surface-atmosphere exchange in a box: Making the control volume a suitable representation for in-situ observations, Agric. For. Meteorol., 255, 68-80, doi:10.1016/j.agrformet.2017.08.037, 2018.
- Pielke Sr, R. A., Mahmood, R., and McAlpine, C.: Land’s complex role in climate change, Phys. Today, 69, 40-46, doi:10.1063/PT.3.3364, 2016.
- Vaughan, A. R., Lee, J. D., Misztal, P. K., Metzger, S., Shaw, M. D., Lewis, A. C., Purvis, R. M., Carslaw, D. C., Goldstein, A. H., Hewitt, C. N., Davison, B., Beevers, S. D., and Karl, T. G.: Spatially resolved flux measurements of NOx from London suggest significantly higher emissions than predicted by inventories, Faraday Discuss., 189, 455-472, doi:10.1039/c5fd00170f, 2016.
- Vaughan, A. R., Lee, J. D., Shaw, M. D., Misztal, P. K., Metzger, S., Vieno, M., Davison, B., Karl, T. G., Carpenter, L. J., Lewis, A. C., Purvis, R. M., Goldstein, A. H., and Hewitt, C. N.: VOC emission rates over London and South East England obtained by airborne eddy covariance, Faraday Discuss., 200, 599-620, doi:10.1039/c7fd00002b, 2017.
- Xu, K., Metzger, S., and Desai, A. R.: Surface-atmosphere exchange in a box: Space-time resolved storage and net vertical fluxes from tower-based eddy covariance, Agric. For. Meteorol., 255, 81-91, doi:10.1016/j.agrformet.2017.10.011, 2018.