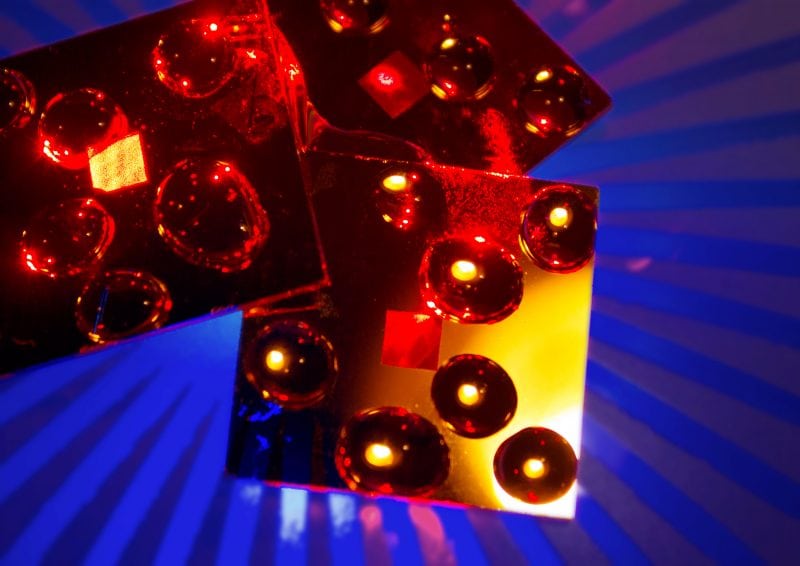
Surface-enhanced Raman spectroscopy (SERS) is a kind of powerful spectroscopic analytical technique which can detect the molecular arrangement, intermolecular interaction, and molecular structure on the interface. This technology is dominantly dependent upon the intensive electromagnetic field in the vicinity of metal or metal-decorated nanostructures due to their localized surface plasmonic resonance, which can dramatically amplify Raman scattering signals (distinct signal of the molecular) of target molecules near the nanostructures.
Due to the high sensitivity and high resolution, SERS is very likely to be utilized in developing advanced biosensor and chemical sensors to realize living cell image, in vitro and in vivo diagnosis, food analysis, environmental monitoring, single molecular detection and so on. However, for practical applications, one of the key issues is to develop facile, low-cost methods to construct large-area SERS substrates with high density and uniform plasmonic nanostructures, which can simultaneously ensure the high sensitivity and reproducibility of the SERS signals.
To resolve this problem, Li et al. have designed a facile and rapid process — one-step voltage-variation anodization of Al foils followed by Ag nanoparticle deposition to low-cost fabricate hybrid nanotip and nanopore arrays (NTNPAs) at large area (5 cm×5 cm). This method is simple, rapid, and inexpensive; it only needs 1560 s and very simple and inexpensive equipment to fabricate uniformly distributed alumina NTNPAs at large area, which are very promising to be evolved to an industry-compatible process. As shown in Figure 1, aligned nanotips with diameters of 42 ± 3 nm and heights of 253 ± 36 nm are uniformly distributed on the whole surface, which shows black-red color. The nanotips can excite intensive electromagnetic field.
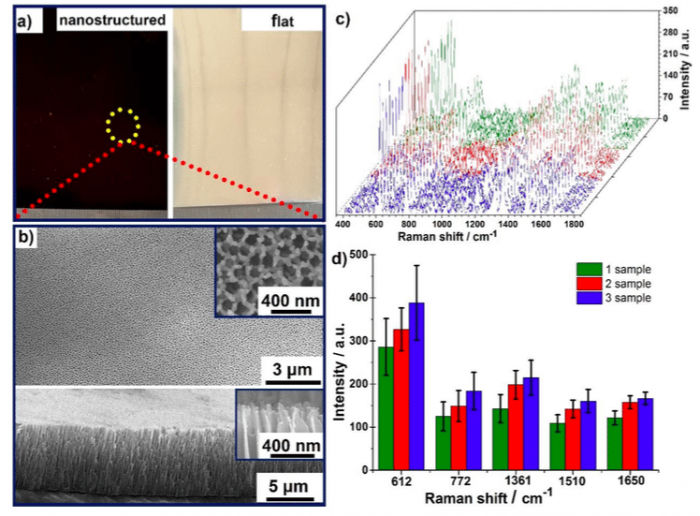
Figure 1. a) Optical images of as-prepared nanostructured sample (left, black-red color) and contrast flat sample (right, like mirrors), which were all deposited with Ag nanoparticles for 400 s; b) SEM top-view (top) and side-view (bottom) of the as-synthesized nanostructured sample in (a). The inset showing the magnified details of top-layer nanotips; c) SERS spectra of 1 μM R6G absorbed on 3 identical nanostructured samples, and each one was randomly chosen 8 spectra; d) The standard deviation and average value of the five prominent peaks at 612, 772, 1361, 1510 and 1650 cm-1, respectively, which obtained from 3 identical nanostructured samples and 30 spots each. (Republished with permission from Elsevier)
Figure 1c shows eight representative SERS spectra of absorbed 1 µM R6G on one nanostructured sample and points from three samples of different batches. No changes were observed in the characteristic peaks compared with the spectra from the different samples, indicating that the SERS signals from different spectra were all of comparable intensity. Figure 1d shows the standard deviation and average value of the five peaks at 612, 772, 1361, 1510 and 1650 cm-1. The relative stand deviation of the intensity at 1650 cm-1 was estimated to be 11.28% for one sample and 11.83% for different samples. The homogeneous distribution of the Raman intensity strongly supports the notion that NTNPAs are uniformly distributed in a large area, which can generate SERS signal with good uniformity and reproducibility.
Another issue for SERS is that it needs an efficient way to bring the target molecules to the surface of plasmonic nanostructures. For dilute aqueous solutions, nanostructures always need to be dipped into the solution for more than 8 h before detection to achieve the uniform absorption of the analyte. It is because the diffuse rate of analyzed molecules to nanosurface is very slow (ca. 60 h/cm). SERS and superhydrophobic functional substrates give a way to resolve the problem. As the nanostructure not only can act as hot spots for SERS detection but also enrich the analyte of a spherical droplet into a very small area due to the condensation effect. To simultaneously achieve this two functions above, it needs to skillfully design the nanostructure. In principle, hybrid NTNPAs could realize the superhydrophobic function after modified with low-free-energy fluorosilane (FS). It is because the nanotips can avoid the capillary effect due to the interrupted cell wall, and the nanopores can provide a stable air-pocket to ensure superhydrophobic property.
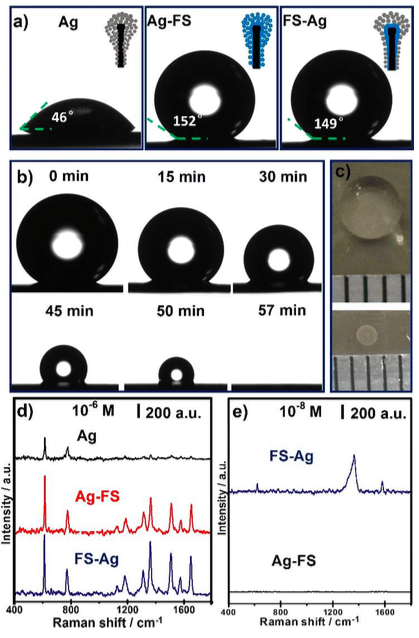
Figure 2. a) Optical images of a 4-μL water droplet on the alumina NTNPAs with Ag nanoparticle deposition (Ag), Ag nanoparticle deposition followed by FS modification (Ag-FS) and FS modification followed by Ag nanoparticle deposition (FS-Ag), respectively; b) The morphology evolution of a 10-μL droplet during evaporation on the FS-Ag nanosample under ambient conditions; c) Photographs show a 20-μL droplet of 1 μM R6G solution (top) and the corresponding concentrated spot (bottom) after evaporation on FS-Ag nanosample; d) SERS spectra of dried 1 μM R6G droplet on Ag, Ag-FS and FS-Ag nanosamples, respectively; e) SERS spectra of dried 20-μL R6G droplet with concentration of 10-8 M on FS-Ag and Ag-FS nanosample, respectively. (Republished with permission from Elsevier)
As seen in Figure 2a, the water static contact angle of NTNPAs with Ag deposition (Ag), Ag deposition followed by FS modification (Ag-FS) and FS modification followed by Ag deposition (FS-Ag), are 46º, 152º, and 149º, respectively. The morphology evolution of a 10-μL droplet during evaporation on FS-Ag sample was recorded (Figure 2b). Before 50 min, due to the low adhesion force between the drop and the surface, the drop reduced in volume while maintaining its quasi-spherical shape (self-similar geometrical transformation), which made the droplet solution became more and more concentrated. After that, the droplet collapsed due to reach a condition of instability, and then continuously and completely evaporated in a confined region.
Figure 2c intuitively shows that a 20-μL droplet of 1 µM R6G solution can be concentrated into a circle spot with a diameter of 1 mm on the FS-Ag sample. Thus, the SERS intensity at 612 cm-1 of a directly dried 10-6 M R6G droplet (20 μL) on FS-Ag nano sample was approximately 3 times higher than that on an unmodified sample (Figure 6d). When the R6G concentration of the droplet further decrease to 10-8 M (Figure 6e), the characteristic peak of R6G at 612, 1361 and 1574 cm-1 still can be clearly detected on the FS-Ag nano sample. Thus, NTNPAs can realize the detection of trace molecular in a space-saving and time-saving way.
These findings are described in the article entitled Facile Fabrication of Superhydrophobic Hybrid Nanotip and Nanopore Arrays as Surface-enhanced Raman Spectroscopy Substrates, recently published in the journal Applied Surface Science. This work was conducted by Juan Li and Yuxin li from Shaanxi Normal University.