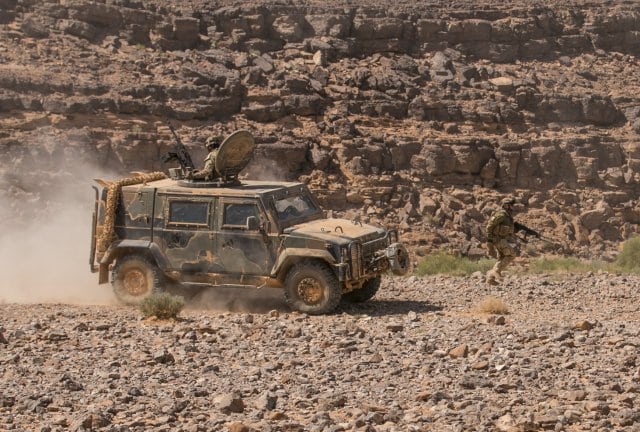
When a spacecraft runs in space, the surface temperature of the sunny side could reach hundreds of degrees. On top of that, the atmospheric friction creates even higher temperatures during the recovery or launch. When an armored car runs on a battlefield, the high temperature caused by the exhaust gas of the engine could reach several hundreds of degrees, and this intense infrared radiation makes it easier to be identified.
When a nuclear power plant runs, the pipe temperature reaches hundreds of degrees, which can lead to accidents or reduce the energy efficiency, if the necessary measures are not taken. All these problems above could be solved with a thermally insulating material.
Thermal conductivity is the most important property of thermal insulating materials, and the lower the thermal conductivity is, the better the adiabatic performance. With the working temperature increased in these application areas, the thermal conductivity has to be measured under high-temperature conditions.
The guarded hot plate (GHP) method is widely considered as the most reliable method to measure the thermal conductivity of insulating material due to its low uncertainty. However, the measurement uncertainty increases as testing temperature rise, making the influence of high-temperature conditions on the measurement results not quite clear.
To analyze the changes of measuring components under high-temperature conditions, a high-temperature GHP apparatus and a low-density mullite specimen with great thermal stability were studied.
By forming a stable temperature distribution around the specimen, the heat from the meter plate heater should vertically transfer from the hot side to the cold side of the specimen, without heat exchange in the plane direction. After the temperature gradient on the specimen reaches a stable state, the thermal conductivity of the specimen should be calculated with the heat from the metering plate, the thickness of the specimen, the meter area and the temperature difference across the specimen measured. Therefore, after the relations between these four components and the high testing temperature were investigated, the calibration methods could be proposed to modify the measuring results of these components, and the assessed uncertainty should be more reliable.
Ideally, all the heat from the metering plate should be vertically transferred to the cold side without heat loss or gain. However, inevitable heat loss occurs, that is not through the specimen to the cold side. Through monitoring the temperature difference between the metering plate and primary guarded plate, this part of heat could be shown, and it increased with the increase of testing temperature.
It is difficult to measure this part of heat quantitatively since mechanization of the heat loss is not clear. Therefore, a calibration method to eliminate the heat loss through controlling the temperature difference between the metering plate and guarded plate instead of calculating the heat loss was proposed. The temperature differences should be recorded at corresponding temperatures and used as bias value to modify the heat flow. During the routine measurements, the metering plate heater would stop increasing output when the temperature of the metering section was the bias value lower than that of the primary guarded hot plate at the corresponding temperature, but not equal to each other. The sum of the heat loss and the heat through the specimen should be the heat in an ideal condition which was measured directly by the power meter.
After the heat flow was calibrated, the thermal expansion of the hot and cold plate was measured and used to calibrate the thickness measurement results. The thermal expansion of meter area and temperature distribution in the plane direction was investigated.
With all the calibrations at high temperatures on these four components finished, the measurement uncertainty at all testing temperature could be assessed. The expanded uncertainty (k=2) increased with the increase of testing temperature and reached 3.2% at 500℃.
These findings are described in the article entitled Calibration Method and Uncertainty Assessment of a High-Temperature GHP Apparatus, published in the journal International Journal of Thermophysics. This work was led by Kai Yao from Shandong Nonmetallic Materials Institute.