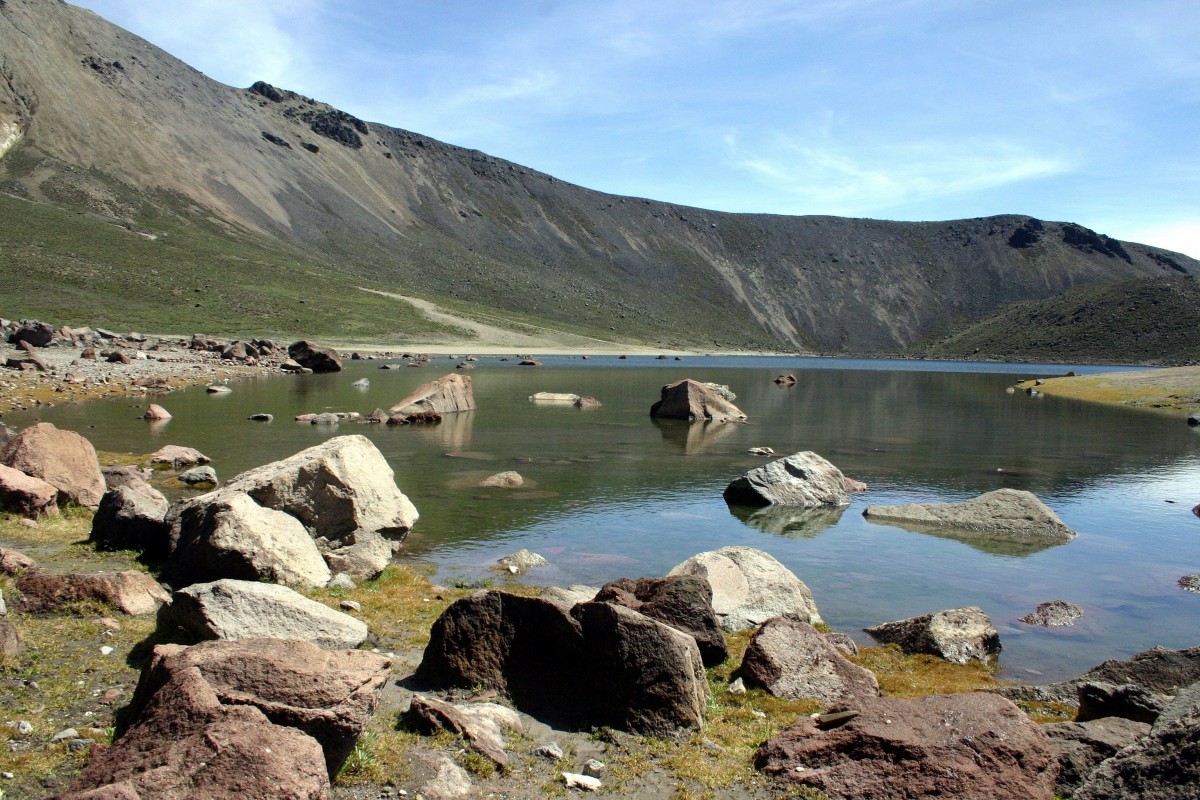
Rare earth element (REE, lanthanides plus yttrium) behavior in natural environments has been extensively studied. REEs are valuable as components of electronic and renewable energy technologies. The European Community lists REEs as critical raw materials. An understanding of the mobility, accumulation, and global cycle mechanisms of REEs in the natural environment is important for evaluating their reserves for future uses and to anticipate environmental issues involved with the exploitation of these metals.
REE extraction and associated critical elements using in‐situ leaching techniques have been optimized to enhance their extraction rates. Dissolved REEs have been used as a proxy for understanding many geodynamic processes, such as magmatic melting and water‐rock interactions. Dissolved REEs have also been used to trace the environmental impacts of human activity such as shale gas hydraulic fracturing fluid analysis. Eventually, the physicochemical properties of REEs allow for their use as robust proxies for studying low- and high-temperature geochemical reactions.
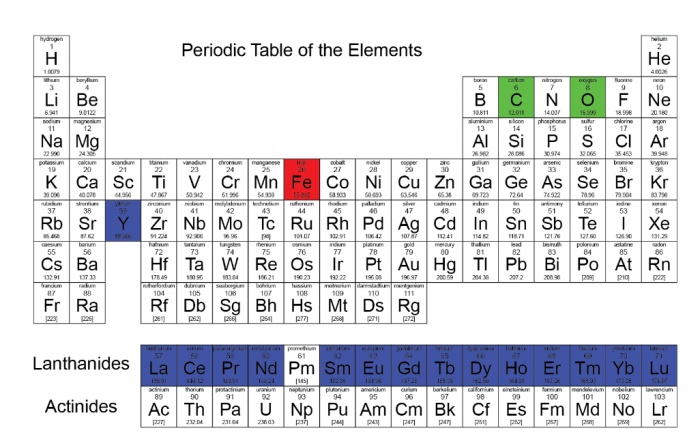
Courtesy of Olivier Pourret
Geological storage of CO2, part of Carbon and Capture and Storage (CCS) technology, has been considered as a potential method for reducing the accumulation of atmospheric greenhouse gases. Geological storage consists of injecting CO2 previously captured from industrial processes into deep underground rock formations. The aim is thereby to permanently remove CO2 from the atmosphere. The effectiveness of storage depends on the reservoir storage sites’ capacity and integrity.
CO2 storage capacity is driven by porosity, reservoir permeability, and the existence of a sufficient impermeable barrier or cap rock in order to contain the CO2 permanently. Several types of geological formations were identified to present suitable capacity for CCS. To date, these identified reservoirs are deep saline aquifers, coal bed formations, oil and gas reservoirs exploited through Enhanced Oil Recovery techniques using CO2, and, finally, depleted oil and gas reservoirs. Among these storage sites, deep saline aquifers were identified as having the largest storage capacity and worldwide repartition. Once injected, part of the CO2 is physically trapped beneath the impermeable cap rock that acts as a seal, and the remainder of the CO2 dissolves into the aqueous phase. Part of this dissolved CO2 might react with the reservoir rocks to form a new mineral (CO2 mineral trapping), or may remain dissolved into the aqueous phase (solution trapping). Therefore, the understanding of CO2‐water‐rock interaction processes are of primary importance to validate the feasibility and effectiveness of CCS.
To study this phenomena, numerous experiments were performed in laboratory conditions coupled with geochemical models, which offer good accuracy for chemical reaction description but are limited in time and scale. The study of natural CO2 hydrothermal-rich water offers good analogs to study longtime CO2‐water‐rock interaction, but appears limited to describe phenomena with good accuracy. Several field experiments were performed as a test pilot to approach the scale of the CCS site and to identify technical and scientific gaps.
These different experimental works showed that several issues must be resolved. These issues include the environmental risks involved with CCS. Several pilot experiments, in the field and in the laboratory, have suggested the potential risks of releasing dissolved trace metals into subsurface freshwater aquifers. The release of dissolved metals may be caused by CO2-enriched brine leaking from an initial deep storage reservoir and moving toward the surface.
Among other phenomena, the remobilization of trace metals is related to in‐situ pH and redox perturbations involved with CO2 injection or leakage. In particular, CO2 perturbation may involve pH acidification, enhancing dissolution of the reservoir rock, generating the release of trace metals that may be initially present in the reservoir rock minerals or sorbed onto mineral surfaces. Understanding the fate and of trace metal elements in subsurface geological environments is important. These issues are also valuable for other geoengineering applications such as toxic waste disposal, long-term underground storage of radioactive waste, and surveys of waste landfills.
The CO2‐water‐rock interactions are driven by the crystallization of newly‐forming minerals at the fluid‐rock interface. Then, part of the feasibility of the CO2 storage is a surface‐driven process, and REEs represent a promising geochemical tool for describing processes occurring at the interface between injected fluids and host rock. The REE behavior in groundwater may help to understand the reactions occurring at the water‐rock interfaces.
Dissolved REE concentration in groundwater is controlled by (i) release of chemical elements involved with weathering processes; (ii) groundwater pH and redox; (iii) sorption processes; (iv) potential complexing of ligands like carbonates in groundwater; and (v) physical hydrogeological factors. Studies of dissolved REE behavior within geothermal fluids and natural CO2-rich water sources have already produced useful results. A fractionation of the REE spectrum occurs during CO2 water and rock interaction processes, and a strong relationship between dissolved REE and water pH has been identified.
The use of REE as tracers of high salinity brine leakage detection in CCS applications has been studied under laboratory conditions. However, several issues were highlighted, such as high salinity content of the samples, causing analytical problems for traces metals analysis. REE signature in fluid inclusion is also a key parameter to understand the behavior of deep geological fluids. In particular, REE signatures are good proxies for evaluating the physicochemical conditions, fluid mixing, and precipitation processes operating during the genesis of valuable geological resources,
During mineral crystallization, REEs behave as driven by their ionic charge and radius. On the contrary, during aqueous processes, metal ions behave according to their external electronic configuration. Accordingly, the main change in the features of normalized REE concentrations and in the Y/Ho ratio can be recognized at the solid‐fluid interface during rock‐fluid interactions. Among the phenomena effecting the behavior of dissolved REE, the role of scavenging REE by metallic oxide and hydroxide phases (i.e. oxyhydroxides such as iron and manganese oxyhydroxides) appears to be especially important.
The scavenging of REE onto iron and manganese oxides is strongly pH dependent. The scavenging onto an amorphous phase such as ferrihydrite (e.g. Fe(OH)3) appears important due to the large reactive surface area. Use of zerovalent iron nanoparticules for REE recovery from acid mine drainage under laboratory conditions showed their capability for removing REE from solution. Fractionation between Light REE (LREE) and Heavy REE (HREE) occurs during scavenging onto metal oxides. This is mainly driven by dissolved species complexation, particularly with carbonate species. Many studies have evaluated REE complexation with organic and inorganic ligands. The complexation process of REE occurring on mineral surfaces is key to understanding the behavior of dissolved REE patterns in the environment.
Understanding dissolved REE behavior is challenging due to the involvement and interdependence of the phenomena described previously. The aim of this experimental work is to study the potential enrichment of dissolved trace metals in the groundwater perturbed by CO2 and its potential application to CO2 geological storage safety site assessment.
A previous study focused on major chemical elements and trace metal remobilization (Rillard et al., 2014). A second set of samples, collected during the same experiment, is used to study the behavior of dissolved REEs. The aim of this experiment is to quantify the effect of CO2 and pH perturbation of the aquifer on release of dissolved REEs. This work may enhance understanding of the fate and transport of dissolved REEs and associated trace metals into the environment for evaluating the safety of geological CO2 storage. The results may also be useful for other geoengineering applications such as geological storage of radioactive waste and mine site remediation.
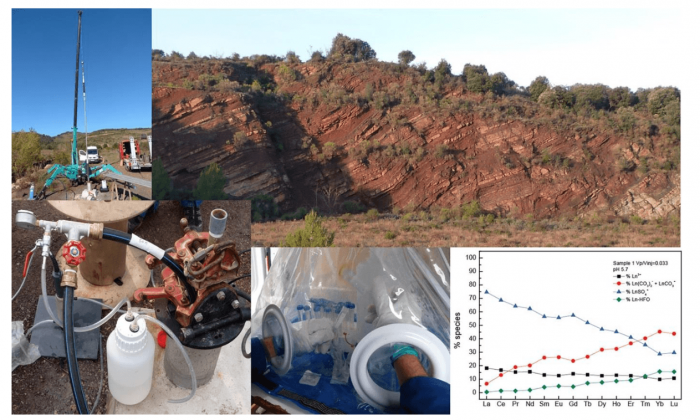
Courtesy of Jean Rillard and Olivier Pourret