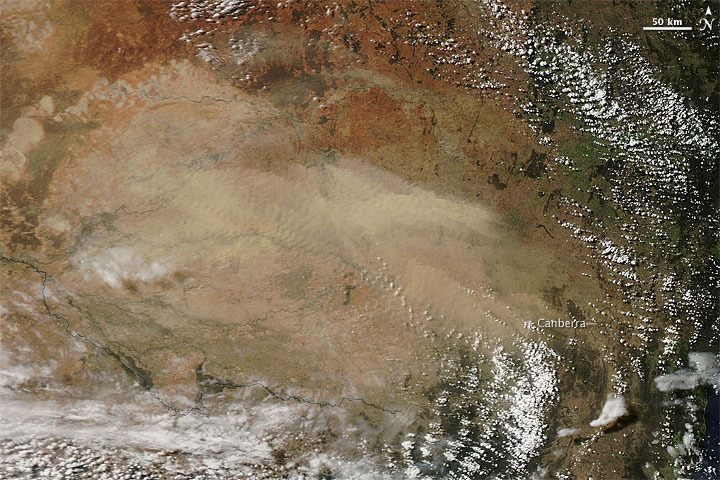
The transport of mineral dust (e.g. soil) through the atmosphere over thousands of kilometers represents an important component of Earth systems. During transport, that is, while in the atmosphere, dust alters radiation exchanges, meaning it affects atmospheric temperature (typically causing a net cooling). Therefore, changes in global dustiness through time are likely to have influenced Earth’s temperature in the past.
In addition, dust influences precipitation by increasing the number of cloud condensation nuclei in the atmosphere. The deposition of dust fertilizes ecosystems with much-needed nutrients. Dust is an important source of nutrients to many environments such as the remote ocean, where it stimulates oceanic phytoplankton production, as well as some tropical rainforests, such as the Amazon. Because primary productivity by phytoplankton results in C02 drawdown from the atmosphere, dust exerts a secondary feedback to temperature, again resulting in cooling.
Overall, therefore, reconstructing dust emission and deposition rates, both now and in the past, provides important information about both climate and biogeochemical processes. However, we still know relatively little about dust transport rates and its role in driving past climate variability; for example, it is hypothesized that that changing dust emissions contributed to tipping the Earth’s climate between glacial and interglacial conditions during the Quaternary Period, although both more records of past dust emissions combined with Earth system modeling are needed to more fully test this idea.
In addition to playing a role in driving changes within the Earth’s systems, dust emission rates also respond to changes in earth surface conditions and climate. For example, increasing aridity may be expected to result in increased dust emissions. For that reason, reconstructing dust deposition rates through time can be used to track changing conditions in dust source areas, such as the hydro-climate state (i.e. wetting and drying) and human land use change (such as changing agricultural practices).
Dust sources and sinks
Dust transport is a significant global sedimentary process. Currently, the major global dust sources are hot arid and semi-arid landscapes, for example, the deserts of North Africa (the Sahara), northwestern China, and Australia, amongst others. In many of these regions, the transport of sediment as dust exceeds river sediment transport. In the past, however, cold climate dust sources, such as glacial outwash plains, were much more important.
Despite the significance of dust, reconstructing dust deposition rates through time is not straightforward. This is because major dust storms which can transport millions of tons of dust over thousands of kilometers will often result in only very thin deposits of dust, albeit over very wide areas. There are some environments, however, in which dust is more easily recognizable, such as within ice sheets or ice fields where dust is often the only source of sediment. Although records of dust deposition reconstructed from ice cores have been extremely valuable, suitable ice fields exist in few locations, that is the Polar Regions, or in alpine environments, both of which may be outside of the major dust transport pathways. Reconstructing dust deposition in other environments can be more complex. Still, records of dust deposition can be constructed from environments such in peat deposits (peat bogs) or from lake sediments.
In some cases, dust may be the only or major contributor of mineral sediment allowing dust to be isolated and analyzed. However, in many cases, catchment-derived sediment transported by rivers is significant. In these environments, the dust component can still be isolated using the application of geochemical fingerprinting techniques to separate dust from other material. The application of geochemical fingerprinting of dust can go much further than separating dust from non-dust; it can also be applied to examining where dust has come from (its source region) and can, therefore, be used to track how different dust source areas have switched on or off through time in response to changes in climate or changes in human land-use.
The geochemical fingerprinting of dust sources works by matching the chemical signature of deposited dust samples with that of its source region. This is possible because different dust source areas have unique geochemical characteristics that reflect the signature of the rocks from which the sediments were derived. This geochemical fingerprint is preserved following the entrainment of sediments by the wind and during its transport and deposition ultimately allowing the source area provenance deposited dust samples to be identified.
Using dust to reconstruct hydro-climate evolution in Australia
Reconstructed records of long-range dust transport-deposition hold significant value for understanding broad-scale environmental change within arid and semi-arid environments. While it has often been assumed that increased dust emissions reflect increasing aridity, our results have shown this is often not the case. Australia is the largest Southern Hemisphere dust source with wind systems transporting dust out Australia to the surrounding oceans and even other landmasses. We have reconstructed rates of Australian dust deposition in alpine peat bogs in New Zealand over the past 8000 years, using geochemical fingerprinting to isolate Australian sourced dust from other material in the bog. These results show Australian dust deposition in New Zealand has averaged 0.6 g/m2/yr (or 6 grams every 10 years) over the past 8000 years, indicating Australian dust contributes significantly to biogeochemical processes in New Zealand.
The results also showed periods of enhanced Australian dust deposition have occurred in response to changing hydroclimate conditions in Australia. For example, during the early-mid Holocene (8,000-4,500 years ago), dust deposition rates were relatively low, with dust sourced largely from semi-arid regions in south-eastern Australia. Importantly, the Lake Eyre Basin, in central Australia, was not active as a dust source during this period. This is despite the Lake Eyre Basin being the largest source of dust from Australia today. Although there are few records for this period from the vast Lake Eyre Basin, the existing evidence implies that Australian monsoon was more active during this period with rainfall spilling over into the Basin, i.e. implying the Lake Eyre Basin may have been too wet to provide dust. After 4,500 years ago, the Lake Eyre Basin switched on as a dust source, implying a major re-organization of Australia’s hydro-climate patterns, to conditions more like today, around this time. This change reflects a generalized drying of central Australia, however, it may also imply increasing climate variability.
Peak Australian dust emissions occurred at around 4,000 to 2,500 years ago, coinciding with records of increased El Nino Southern Oscillation (ENSO) climate variability. We think this is not coincidental, that is, enhanced ENSO activity is marked by switches between wet El Nino events, where the rivers of central Australia flow and bring fresh (fine-grained) sediment to its floodplains and ephemeral lakes. Then, during dry El Nino events, this sediment dries and is transported by the wind. Interestingly, therefore, peak dust emissions over the mid to late Holocene temporally match peak climate variability and imply continual resupply of sediment in source areas results in enhanced dust emissions.
The human dust link
In addition to responding to natural environmental variability, dust emissions may also respond to human activity. The semi-arid Murray-Darling Basin (MBD) in south-eastern Australia is Australia’s major agricultural region, producing 40% of its agricultural products. Alpine peat cores from the Snowy Mountains on the eastern (downwind) edge of the Basin track changing dust deposition following the development of agriculture in the Basin from the 1850’s AD. The peat cores demonstrate that dust deposition rates increased by approximately 4 times following introduction of sheep, cattle, and crop production in the Basin. In this case, increased dust emissions occurred in response to the introduction of hard hooved animals (which break up protective soil crusts), associated vegetation clearing, and water diversion (irrigation), which collectively expose soils to wind erosion.
Major drought events stand out as episodes of enhanced dust deposition in the core. These drought events coincide with dramatic oral reports of dust storms and wind erosion in the Basin between the 1890s and 1940s, a period which has been termed the Australian dust bowl. Importantly, the peat bogs record diminished dust deposition from the 1980s, when improved soil conservation measures and programs were widely initiated in Australia. Consequently, the studied cores appear to highlights the value of concerted management for improved soil conservation.
Understanding the role of dust
Significant progress has been made in understanding changing dust emissions and the role of dust in Earth’s systems over the last few decades; however, much remains to be learned. There are still relatively few paleo records of dust emissions and, consequently, both the controls on dust emissions through time, as well as the contribution of dust to Earth’s systems, remains to be fully understood. In particular, records of dust deposition hold great promise for reconstructing past wind speeds and air-mass circulation patterns. In addition, the contribution of dust deposition to ecosystem fertilization, e.g. via soil formation, remains to be fully explored. It is clear, however, that dust emissions respond sensitively to environmental change and therefore developing records of long-range dust deposition has the capacity to provide vital information on board-scale changes to landscapes.
These findings are described in the article entitled Palaeo-dust records: A window to understanding past environments, recently published in the journal Global and Planetary Change. This work was conducted by Samuel K. Marx and James N. Hooper from the University of Wollongong, Balz S. Kamber from Trinity College, Dublin, Hamish A. McGowan from the University of Queensland, Lynda M. Petherick from the Victoria University of Wellington, Grant H. McTainsh from Griffith University, Nicola Stromsoe from Charles Darwin University, and Jan-Hendrik May from the University of Melbourne.