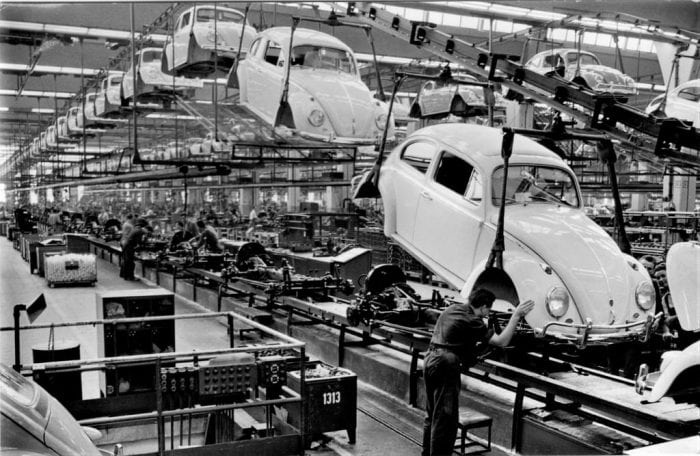
In the early 1900s, Henry Ford revolutionized mass production with the development of the assembly line: a method of combining every step of a production process in a way that optimizes efficiency. Today, metabolic engineers look to apply a similar principle for the mass-production of chemicals with the advent of synthetic microbial consortia.
Metabolic engineering is a relatively new scientific field that utilizes genetic engineering technology to modify the genetic code of organisms to enable the enhanced production of desired chemical and biological products. For example, the group of genes that lead to the production of opioids in the opium poppy plant can be essentially “cut and pasted” into the genome of the common baker’s yeast, Saccharomyces cerevisiae, allowing it to replicate the same biochemical synthesis process [1]. In the future, this concept will hopefully allow for the cheap, safe, and efficient mass-production of chemicals and pharmaceuticals reducing the environmental footprint of chemical production.
However, bacteria and yeast are much less complex than plants. How could a simple, single-celled microorganism ever replace a process carried out by such a complex higher organism? Metabolic engineers believe the answer lies in microbial consortia.
Microbial consortia are an assembly of several different microbes working together to optimize each step of chemical production. Like Ford’s assembly line, this division of labor approach enables a synergy of microbial community members resulting in the completion of more complicated processes than what could be done by a single simple organism. Engineers design a different microbe to carry out each step in a production pathway. For example, the first microbe builds precursor chemical A, which is then transferred to the second microbe, which converts chemical A into chemical B, and so on. This process is ubiquitous in nature, and even humans have been using it for thousands of years in processes like waste degradation and alcohol fermentation.
Natural microbial communities have evolved into consortia to compete and survive as a collective unit. For example, many different types of bacteria and fungi found in soil work together to decompose plant matter and release nutrients. Humans began to use natural consortia in the process of fermenting drinks, such as beer, as early as 5,000 years ago. However, modern-day efforts for using consortia rely heavily on engineering design because the chemicals being produced by metabolic engineers are not natural for bacteria, and the process has not been optimized through evolution.
This method of chemical production has fallen short of economic viability due to its complicated nature. Genetic engineering is a relatively new trade, with many advancements yet to come. In designing synthetic consortia, the main difficulties are related to population dynamics and transportation of metabolites between members of the consortia. Population dynamics describe how the different microbes might interact, such as one population overtaking another. This leads to one population dominating the nutrients found in their environment, leaving the other(s) without enough nutrients to survive.
Furthermore, the transfer of metabolites can hinder the viability of a consortium if not all products/intermediates easily transfer into or out of a cell. This is a very difficult issue because transport processes are oftentimes complex and varied between organisms.
Yet, consortia have a lot of promising outcomes. By using different microbes that are optimized to perform specific steps of each process, microbes work together to significantly increase productivity. The division of labor approach maximizes productivity in the pathway. Recently, the use of a bacterial consortium has allowed for an unprecedented 970-fold improvement in production of a flavonoid nutraceutical, Afzelechin [2].
Additionally, the use of synthetic consortia has enabled the microbial production of the natural red antioxidant pigment from strawberries (Callistephin) and precursors to the anticancer drug Taxol, which had not been previously synthesized from a single microbial culture [3,4]. Genetic optimization of individual strains allows for the scientists to choose microbes best suited for that particular part of the production pathway. Additionally, consortia reduce stress on each individual strain, therefore allowing for more efficient production.
Certain advancements are currently being investigated to improve the previously discussed hindrances. Micro-scale spatial structures are small cage-like devices that can contain microbial cultures in order to control their growth while allowing for thorough mixing of chemical components. These devices could be used to solve the issue of population dynamics by allowing the formation of special niches for the slow-growing strains.
The use of quorum sensing is another possible direction. Quorum sensing is a feature that microbes have evolved, allowing them to sense their population density and respond accordingly. This mechanism is very complex to engineer but eventually could be used to create a growth-regulating cellular response when one population begins to overtake another.
Both advancements could help make co-culture technology a more mainstream way of addressing problems in metabolic engineering. With more research and new developments, consortia-based technology is a promising source of future groundbreaking studies.
The truth is, microbes were never meant to work alone. Through evolution, they have always relied on consortia to compete and survive. This is why current-day metabolic engineers are looking to nature and evolution for ideas in engineering design. Evolution has optimized consortia to maximize productivity and developed methods for population control and chemical transport. Therefore, it is necessary that metabolic engineers take note if they want to design a system that can truly revolutionize methods for the mass-production of chemicals.
These findings are described in the article entitled Use of bacterial co-cultures for the efficient production of chemicals, recently published in the journal Current Opinion in Biotechnology [5]. This work was conducted by J. Andrew Jones and Xin Wang from Miami University – Ohio.
References:
- Galanie S, Thodey K, Trenchard IJ, Filsinger Interrante M, Smolke CD: Complete biosynthesis of opioids in yeast. Science (80- ) 2015, 349:1095–1100.
- Jones JA, Vernacchio VR, Sinkoe AL, Collins SM, Ibrahim MHA, Lachance DM, Hahn J, Koffas MAG: Experimental and computational optimization of an Escherichia coli co-culture for the efficient production of flavonoids. Metab Eng 2016, 35:55–63.
- Jones JA, Vernacchio VR, Collins SM, Shirke AN, Xiu Y, Englaender JA, Cress BF, McCutcheon CC, Linhardt RJ, Gross RA, et al.: Complete Biosynthesis of Anthocyanins Using E. coli Polycultures. MBio 2017, 8:e00621-17.
- Zhou K, Qiao K, Edgar S, Stephanopoulos G: Distributing a metabolic pathway among a microbial consortium enhances production of natural products. Nat Biotechnol 2015, 33:377–383.
- Jones JA, Wang X: Use of bacterial co-cultures for the efficient production of chemicals. Curr Opin Biotechnol 2018, doi:10.1016/j.copbio.2017.11.012.