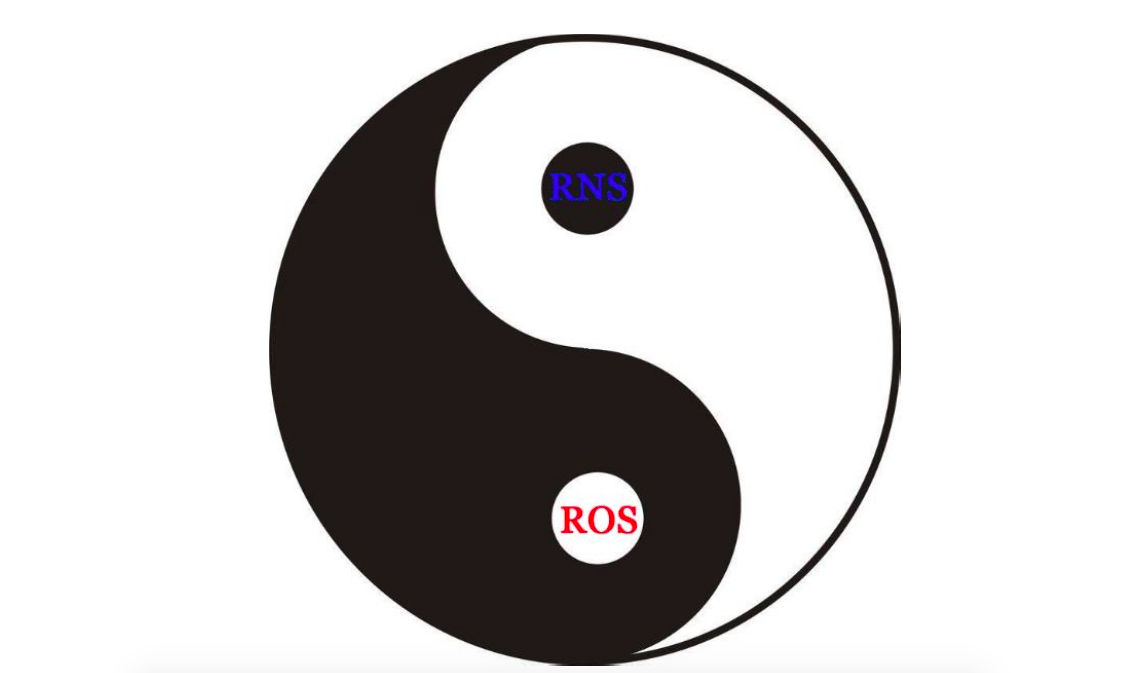
The molecule nitric oxide (NO; alternately named nitrogen oxide or nitrogen monoxide) has been well-known for a long time to be a part of the nitrogen cycle. The 1998 Nobel Prize in Physiology or Medicine was awarded for discovering NO’s role as a cardiovascular signaling molecule since it was proclaimed the Molecule of the Year by the Science journal in 1992.
In the past 30 years, this molecule has become the hub of investigation of many physiological and pathological processes in both animals and plants. The primary reason may due to the fact that, under normal physiological or unfavorable environmental conditions, NO has pleiotropic functions at different levels such as cells, tissues, and organs. Over the years, the attention on NO was only focused on its contribution to atmospheric pollution because it together with nitrogen dioxide constitute the group of nitrogen oxides that affect the air quality and plant growth.
However, in 1987, two independent groups confirmed that NO, designated as an endothelium-derived relaxing factor, was involved in the regulation of vascular vasodilation. It is worth mentioning that Fewson and Nicholas published their first report in 1960 showing that microorganisms and higher plants can use NO as an intermediate in nitrogen metabolism (Fewson and Nicholas, 1960). By the 1990s, studies regarding NO function has been developed to plant cells, which are involved in the mechanisms of interaction with beneficial microorganisms, defense against pathogens, and adverse environmental stress.
On the other hand, with rapid urbanization and industrialization, heavy metal pollution throughout the world has increased unexpectedly and has exacerbated potential risks to human health, especially resulting in disrupting renal and immune functions and even causing cancer. The latest survey showed that soils contaminated with cadmium (Cd) accounted for over 7% of the land according to the National Soil-Environmental Quality Standard of China (NSEQSC GB 15618-1995), making it one of the most serious problems in China. Cadmium, as a non-essential element for plant growth, can disrupt cellular redox homoeostasis to burst the reactive oxygen species (ROS; e.g., superoxide radical, hydrogen peroxide, singlet oxygen, and so on) through ‘Fenton-Haber-Weiss’ reactions, resulting in the activation of oxidative stress in plants, resulting in the generation of oxidative stress. To date, scarce information has been recorded about the relationship between NO and •NO-derived molecules such as S-nitrosoglutathione, S-nitrosoglutathione reductase, and protein tyrosine nitration peroxynitrite (designed as reactive nitrogen species or RNS) and ROS in plant-metal interactions.
Considering these challenges, the principal objective of our study was to conduct the first investigation of the metabolic mechanism of RNS and ROS under Cd stress by using white clover as the studied plant and to improve our understanding of their potential interrelationships. In our study, therefore, three hypotheses were investigated as the following: (i) Cd will provoke nitrosative stress by regulating the levels of NO-derived molecules in Cd-induced white clover plants. (ii) NO-mediated post-translational modifications will compromise the functioning of the ascorbate-glutathione cycle (or named Foyer-Halliwell-Asada pathway) and free amino acids metabolism when Cd leads to oxidative stress. (iii) Both ROS and RNS families are characterized by the rigorous metabolic interaction that will modulate the nitro-oxidative stress response in Cd-induced plants.
According to our research, we found that the reciprocal interactions between the RNS and ROS signaling pathways exist in Cd-stressed plants. On the one hand, Cd causes lipid peroxidation and ROS accumulation, thus provoking oxidative stress. In general, Cd causes ROS burst via three possible pathways: (1) it induces Cd-responsive microRNA expression and then inhibits the function of copper- and zinc-superoxidase dismutase; (2) it blocks the regulatory role of calcium ions; or (3) enhances nicotinamide adenine dinucleotide phosphate (NADPH) oxidases. To respond to the overproduction of ROS, antioxidative systems work for ROS-regulation, such as antioxidant enzymes, NADPH-generating dehydrogenases, and free amino acids.
On the other hand, the peroxynitrite is produced from the reaction as NO and superoxide radical, which is verified as mediating the protein tyrosine nitration. This RNS is considered an effective oxidizer that reacts with carbon dioxide and further breaks down into the powerful nitrating agents carbonate and nitrogen dioxide under physiological conditions. In this regard, RNS can change the target proteins via post-translational modifications with S-nitrosylation and tyrosine nitration. Simultaneously, •NO can react with reduced glutathione to produce S-nitrosoglutathione, which, through a process of trans-nitrosylation, affects other peroxisomal enzymes. The results provided a comprehensive metabolite evidence that clearly confirms the relationships between ROS and RNS in Cd-stressed plants, supporting their regulatory roles in response to nitro-oxidative stress and providing an in-depth understanding of the interaction between two families subjected to metal stresses.
These findings are described in the article entitled Signalling cross-talk between nitric oxide and active oxygen in Trifolium repens L. plants responses to cadmium stress, recently published in the journal Environmental Pollution. This work was conducted by Shiliang Liu, Rongjie Yang, Xi Li, Mingyan Jiang, Bingyang Lv, Mingdong Ma, Qibing Chen from the Sichuan Agricultural University and Durgesh Kumar Tripathi from the Motilal Nehru National Institute of Technology Allahabad.
Cover photo: A Chinese traditional Yin and Yang diagram showing the interaction between RNS and ROS. In Chinese philosophy, Yin and Yang describe how seemingly opposite or contrary forces may actually be complementary, interconnected, and interdependent in the natural world, and how they may give rise to each other as they interrelate to one another.