
Accidentally touching your hot oven rack makes you painfully aware that metals are very good at conducting heat. If we could zoom in to the atomic level, we’d see that tiny electrons are to blame. The same reason why electricity can freely flow down copper wires also explains why electrons are equally well suited to conduct those hundreds of degrees of oven heat straight into your finger’s nerve endings. And to think that a thin silicone oven mitt with its inferior thermal conductivity would have easily saved you from this thought-provoking pain.
This microscopic view of the important role of conduction electrons for the transport of heat in metals goes way back. Indeed, the transport of heat in solids is one of the oldest problems in physics, dating back to the earliest formulations of thermodynamics. In 1822, Joseph Fourier first formulated the classical law of heat conduction, describing how heat flows from the hot end of a material to the cold one. Nearly a decade later, in 1905, Albert Einstein famously proved that this type of macroscopic transport can be derived from the random motion of microscopic particles.
Many decades of research since then have contributed to a microscopic description of heat, greatly advancing the understanding of the (quantum) nature of heat carriers. We now know that heat in solids is carried by excitations of the atomic lattice, known as phonons, as well as by free conduction electrons. The classical law of heat conduction is still used today to describe thermal transport in all but the most extreme cases.
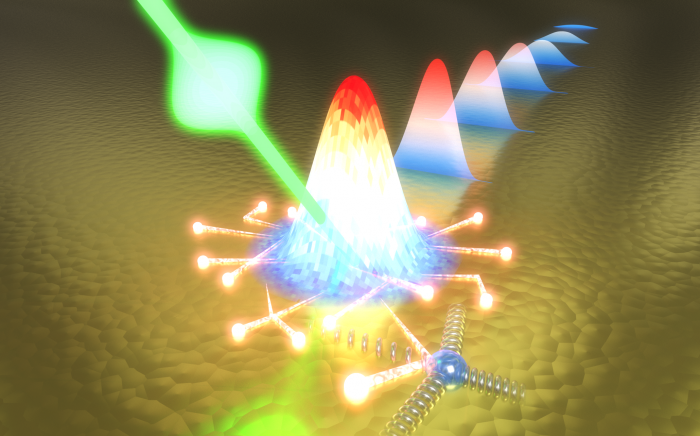
Optically excited hot electrons in a gold film can be imaged as they diffuse and scatter with lattice vibrations.
Credit: Florian Sterl (Sterltech Optics) & Alexander Block (ICFO)
More recently, scientists have found out that this classical law breaks down when the heating happens on incredibly fast time scales. When light pulses with a duration of less than a trillionth of a second (< 1 picosecond) shine on a metal, the absorbed energy gets transferred to the electrons, leading to a situation of local non-equilibrium, where Fourier’s law needs to be revised. While the atomic lattice stays relatively cool, the electrons are heated up to thousands of degrees. These extremely hot electrons do not cause the metal to melt, however. That is because this high temperature only lasts for a very short time. After a few more picoseconds, the metal’s temperature ends up only tens of degrees above room temperature. So where did all this heat go?
To answer this question, scientists from the Institute of Photonic Sciences (ICFO) in Spain developed a microscope which is capable of tracking hot electrons by capturing videos with unprecedented simultaneous spatial and temporal resolution. By illuminating thin gold films with two spatially and temporally offset laser pulses, they were able to locally heat the gold film, and then watch as the hot electrons moved out of the illuminated area. They use a kind of super-resolution technique to watch the electrons move beyond the classical limits of microscopy, with nanometer and femtosecond resolution. The results, published in the journal Science Advances, show that the diffusion of heat in thin gold films happens in two distinct steps.
The scientists visualized for the first time that hot electrons diffuse 100-times faster than the ordinary thermal diffusion within the first few picoseconds after the laser pulse hits the gold sample. Then, they observe a transition to the classical diffusion regime as predicted by Fourier’s law. The researchers, in collaboration with the Ben-Gurion University of the Negev in Israel, were able to explain the results with the help of a full three-dimensional simulation of the thermo-optical response. Due to the simultaneous spatial and temporal resolution, this experiment allows the direct distinction between the two competing hot-electron cooling mechanisms of diffusion and electron-phonon coupling, which was not possible in previous experiments.
This work has implications both in fundamental science as well as for technological applications. This regime of non-equilibrium thermodynamics has already started to spark new avenues in the theoretical description of heat transport at the nanoscale. Furthermore, these effects could potentially be used to improve the efficiency of solar cells, where the light energy needs to arrive at a charge extraction region before being lost. It has been predicted that by harvesting the generally unused extra energy of these fast, hot electrons the efficiency could be significantly boosted. Similar advances will help to design the next generation of ultrafast light detectors.
These findings are described in the article entitled Tracking ultrafast hot-electron diffusion in space and time by ultrafast thermomodulation microscopy, recently published in the journal Science Advances.