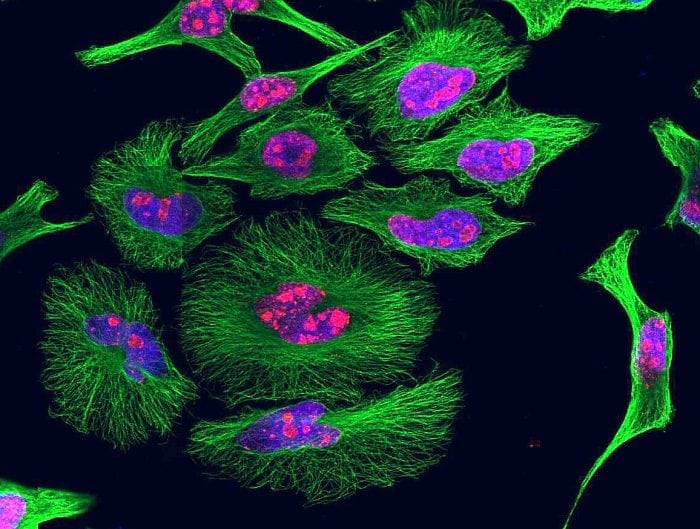
A healthy adult human has about 2-3×1013 red blood cells (RBC) with a hemoglobin (Hb) content of about 3.108 molecules/RBC, equivalent to ~109 heme molecules per RBC. Clinically silent intravascular hemolysis can lead to the release of relatively high levels of biologically active Hb into plasma. The prosthetic heme group of extracellular Hb can be released upon oxidation, giving rise to labile heme (1-3).
When released from Hb however, heme is most probably never in a free form and instead becomes loosely bound to plasma macromolecules and low molecular weight ligands in a metabolically (redox) active form (4, 5). Labile heme acts in a pro-oxidant, cytotoxic, pro-inflammatory and vasoactive manner (6), presumably fostering the pathogenesis of disease associated with more or less severe hemolysis, such as sickle cell disease (7, 8), malaria (1, 3, 9) or severe sepsis (10).
Given the current limitation in methodologies allowing the accurate quantification of labile heme in plasma, it has been difficult, however, to pinpoint unequivocally the relative contribution of labile heme to the pathogenesis of these hemolytic conditions. To overcome these limitations we generated a panel of heme-specific single domain antibodies (sdAbs) with the aim of achieving a better characterization of released heme during hemolysis (5).
We found that heme-specific sdAbs can recognize labile heme specifically but with a low affinity typically in the range of 10-7 M (5). Of note, the affinity of these sdAbs towards heme is lower than that of physiologic plasma heme scavengers, such as hemopexin, high- or low-density lipoprotein or even albumin (KD>10-7 M). As such the heme-specific sdAbs recognize heme only if not bound to plasma molecules with an affinity >10-7 M (5).
Using Spectrophotometry and Resonance Raman we established that the heme-specific sdAbs interact specifically with heme via a cysteine acting as the fifth ligand for iron and possibly a lysine or a tyrosine acting as the sixth axial iron ligand. Using Fourier-Transform Infrared Spectroscopy (FTIR) analyses we also established that heme-specific sdAbs undergo a conformational change in the anti-parallel β-sheets, presumably to accommodate heme (5).
Using a combination of bioassays we determined that about 2-8% (~2-5 µM) of the heme released in plasma during different experimental hemolytic conditions in mice is (bio)available for cellular internalization (5). At this labile heme acts in a pro-oxidant, cytotoxic, pro-inflammatory and vasoactive manner, supporting the notion that labile heme participates in the pathogenesis of hemolytic conditions. Our study also proposes that this or other heme-specific sdAbs maybe be of therapeutic use as they can neutralize the pro-oxidant activity of labile heme in vitro (5). Whether this also occurs in vivo, remains, however, to be tested experimentally.
As a final note heme-specific sdAbs can be used to visualize intracellular heme by immunostaining as well as detect intracellular heme by flow cytometry, revealing that the majority of the cellular heme is contained in the mitochondria with a minor portion detected in the endoplasmic reticulum (5). We cannot exclude, however, that under the experimental conditions used there is no release of heme from intracellular proteins.
References
- Pamplona, A., A. Ferreira, J. Balla, V. Jeney, G. Balla, S. Epiphanio, A. Chora, C. D. Rodrigues, I. P. Gregoire, M. Cunha-Rodrigues, S. Portugal, M. P. Soares, and M. M. Mota. 2007. Heme oxygenase-1 and carbon monoxide suppress the pathogenesis of experimental cerebral malaria. Nat Med 13: 703-710.
- Hebbel, R. P., W. T. Morgan, J. W. Eaton, and B. E. Hedlund. 1988. Accelerated autoxidation and heme loss due to instability of sickle hemoglobin. Proc Natl Acad Sci U S A 85: 237-241.
- Ferreira, A., I. Marguti, I. Bechmann, V. Jeney, A. Chora, N. R. Palha, S. Rebelo, A. Henri, Y. Beuzard, and M. P. Soares. 2011. Sickle Hemoglobin Confers Tolerance to Plasmodium Infection. Cell 145: 398–409.
- Yuan, X., N. Rietzschel, H. Kwon, A. B. Walter Nuno, D. A. Hanna, J. D. Phillips, E. L. Raven, A. R. Reddi, and I. Hamza. 2016. Regulation of intracellular heme trafficking revealed by subcellular reporters. Proc Natl Acad Sci U S A 113: E5144-5152.
- Gouveia, Z., A. R. Carlos, X. Yuan, F. Aires-da-Silva, R. Stocker, G. J. Maghzal, S. S. Leal, C. M. Gomes, S. Todorovic, O. Iranzo, S. Ramos, A. C. Santos, I. Hamza, J. Goncalves, and M. P. Soares. 2017. Characterization of plasma labile heme in hemolytic conditions. FEBS J 284: 3278-3301.
- Soares, M. P., and M. T. Bozza. 2016. Red alert: labile heme is an alarmin. Curr Opin Immunol 38: 94-100.
- Belcher, J. D., C. Chen, J. Nguyen, L. Milbauer, F. Abdulla, A. I. Alayash, A. Smith, K. A. Nath, R. P. Hebbel, and G. M. Vercellotti. 2014. Heme triggers TLR4 signaling leading to endothelial cell activation and vaso-occlusion in murine sickle cell disease. Blood 123: 377-390.
- Schaer, D. J., P. W. Buehler, A. I. Alayash, J. D. Belcher, and G. M. Vercellotti. 2013. Hemolysis and free hemoglobin revisited: exploring hemoglobin and hemin scavengers as a novel class of therapeutic proteins. Blood 121: 1276-1284.
- Ferreira, A., J. Balla, V. Jeney, G. Balla, and M. P. Soares. 2008. A central role for free heme in the pathogenesis of severe malaria: the missing link? J Mol Med 86: 1097-1111.
- Larsen, R., R. Gozzelino, V. Jeney, L. Tokaji, F. A. Bozza, A. M. Japiassu, D. Bonaparte, M. M. Cavalcante, A. Chora, A. Ferreira, I. Marguti, S. Cardoso, N. Sepulveda, A. Smith, and M. P. Soares. 2010. A central role for free heme in the pathogenesis of severe sepsis. Sci Transl Med 2: 51ra71.
The study, Characterization of plasma labile heme in hemolytic conditions was recently published in The FEBS Journal.