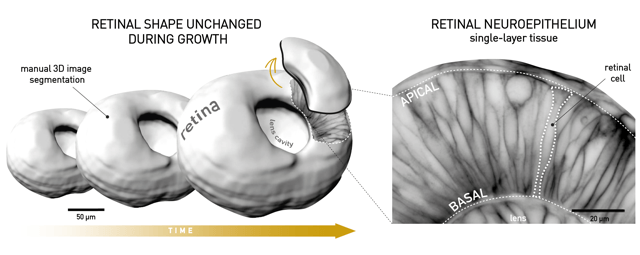
During the development of an organism, all the different tissues take their shape and grow. Ultimately, they all together form a functional adult. The big motivation and task of developmental biologists are to understand how during this process tissues get formed by their building blocks, the cells.
Scientists are still trying to uncover how single cells need to collectively act to form the shape and structure of, for example, the skin, bone, brain or the eye. Many studies have looked at this formation of tissue shapes, which happens during the process of morphogenesis. In fact, throughout the history of developmental biology, as well as today, the research focus of many developmental biologists has been the emergence of shapes and shape change. Despite the indispensable insights these studies gave us, they did not assess an equally important aspect, which is the maintenance of shape. More specifically, it had still not been shown what cells need to do to prevent changes in shapes of tissues as tissues grow.
Many tissues, such as the human ear or eye, establish their shape very early on during development. After becoming tubes, sheets or cups, these tissues then have to grow and mature, but all while keeping their shape unchanged. As an example, you can imagine an entire baby human growing into its adult self; you yourself grew from ~50 cm to ~170 cm long (on average). Still, during this process, your palms kept their shape, both of your ears grew and your eyeballs stayed round. This might seem like it was a simple task for your cells as they were just supposed to not change the already set shapes.
However, as tissues grow, the physical and chemical conditions around them change, they become more squished, stretched and need more food, for example. It is not at all clear which exactly these changing conditions are at different times and for different tissues nor how they might affect collections of cells. For this reason, it is not trivial to predict how properties of cells such as their division, shape or size work together to keep tissues in shape in a changing environment. Despite the importance of this question, tissue shape maintenance during growth was not at all explored so far.
We have tackled this problem in our recent publication in PLoS Biology (Matejčićet al., 2018). Here, we showed how single cells allow the tissue of the vertebrate retina to grow isotropically and keep its smooth shape while it becomes bigger and bigger. I performed all experiments for this study in the lab of Caren Norden at the Max Planck Institute of Molecular Cell Biology and Genetics in Dresden, Germany. The theoretical work was done by our collaborator from The Francis Crick Institute in London, UK, Guillaume Salbreux.
Why would anyone study the growth and shape of the retina in the first place? The vertebrate retina grows out of the neural tube and is a part of the central nervous system (CNS). Therefore, it might give us more general insights, applicable to other parts of the CNS. In addition, the retina is a very important tissue. When mature, the neuronal retina collects the light impulses that pass through it and transmits them to the visual parts of the brain via the optic nerve, allowing us to see. As it lies directly in the light path of the vertebrate body, the correct shape of the retina is essential in order for the light to propagate through it in the best possible way. This is evident in human conditions such as the macular pucker, where even small glitches in the smooth shape of the retina, such as wrinkles and thickenings, cause people to have distorted vision. A healthy vertebrate retina has the shape of a smooth hemispherical cup. This cup is already established early in embryogenesis and then needs to be kept in shape as the tissue grows. The cup shape is geometrically very simple, so we can easily detect and analyze and disturbances in it. All these characteristics make the retina a great system to study the question of shape maintenance.
We have analyzed the maintenance of the cup shape of the retina using the zebrafish, a small model vertebrate organism, whose embryo, in addition to having retinal structure and shape strikingly similar to humans, is transparent and develops fast ex utero. Because of these features of the zebrafish retina, in particular, we could study the retinal cup shape and its single cells during the entire phase of proliferative growth in single 24-hour imaging sessions. In my analysis, I first imaged the zebrafish embryos and manually segmented out their retinal cups in 3D from microscopy images. From this, we learned that the zebrafish retinal cup grows from ~1×106µm3to ~4 x106µm3in ~24 hours. This also revealed how fast retinal progenitor cells divide (~ every 6 hours) and that they, interestingly, become smaller and smaller (from ~440 µm3to 140 µm3) as the whole tissue gets bigger. During the first phase of growth, all retinal progenitor cells divide, and later on, they have a 35% chance to exit the cell cycle and become neurons. Together, this showed us how the retina grows over development by adding more cells to the tissue through cell division.
So, more and more cells in the retina increase retinal size over time. But in order to also keep retinal shape unchanged during growth, these new cells need to be added throughout the tissue in a very coordinated way, one that is uniform and will not cause the tissue to grow more on one side than on another. Indeed, this is exactly what happens in the retina, and we’ve shown that cell divisions in it do not orient along a preferred axis and that their distribution throughout the tissue is uniform. This means that retinal proliferation allows homogeneous, isotropic tissue growth. Is this all there is to it, though? Can cells just keep being added to the retina uniformly and the shape will grow unchanged?
If we think about the question of shape in general, it becomes clear that in order to maintain shape, the width and the height of an object need to increase in concert. In other words, its aspect ratio needs to be kept constant. Indeed, if we quantify the aspect ratio of the retina during development, we see that the retinal surface-to-thickness ratio does not change as the retina grows. The retinal tissue is a thick, single-layered epithelium. The cells that make it up are tightly packed and each attached at both the outer (apical) and the inner (basal) surface of the retinal cup. This means that as the retina becomes thicker during growth, its cells need to elongate to keep its aspect ratio unchanged. In our study, we showed that this is exactly what happens in this system. The already long, slender retinal cells thin-out and elongate even further.
During this process of elongation, a subcellular component, the protein actin, redistributes inside retinal cells. In retinal cells, actin gets depleted from the long lateral cell-cell interfaces and stays only at the tops and bottoms of cells. The actin meshwork is already well known to affect cellular tension and shape in tissues in dependence of its distribution, structure and its active contractions governed by the cellular motor myosin. We assumed that the more actin the cell has at its membranes, the higher the mechanical tension will be at this part. In these terms, we devised a physical model in which the depletion of actin from the lateral cell membranes, like the one we observed in the retina, would lower the lateral tension in the cells. Such theoretical change in the distribution of tension recapitulated our experimental measurements of the lateral elongation of retinal cells and increase in retinal thickness and, overall, could explain the maintenance of the retinal aspect ratio during growth.
In addition to theoretical modelling, we have further tested our hypothesis that actin redistribution enables constant retinal aspect ratio during growth. Using a zebrafish mutant in histone-deacetylase 1 (hdac1), we have shown that if actin remains rich at the lateral cell membranes, retinal cells do not elongate. This changes retinal shape, and the retina initially grows with the same increase in the outer surface, but thinner. With more and more cells added to this mutant tissue during growth, the “thin” retinal cups start breaking their smooth surface and folding into themselves. Folds start forming throughout the cup tissue and result in a wrinkled structure more resembling a miniature folded brain than a retina.
We have also transplanted mutant cells into the wildtype retina and vice-versa. In this experiment, we observed that transplanted cells behave like their new host tissue, rather than as themselves in terms of actin distribution and elongation. This meant that cells are instructed by their environment to redistribute actin from the lateral cell-cell interfaces and that this is a non-cell-autonomous event. In earlier stages of retinal growth, before retinal cells elongate, but also in the short-celled hdac1 mutant, the maintenance of the prominent actin on the lateral cell membranes is dependent on an intact extracellular matrix. This meshwork of molecules underlies the retinal epithelial tissue as the thin basal lamina to which cells attach to and allows them to respond to their environment. Among other things, it can affect the actin distribution inside cells and cellular tension. Therefore, a certain change in the composition of the extracellular matrix at the right time during development might happen and allow retinal cells to redistribute actin, elongate and ultimately prevent retinal shape from changing during growth.
Overall, this study is the first to investigate how tissues keep their shape unchanged while they grow, in vivo. We identified a seemingly simple change in cell shape, coordinated throughout the tissue, that enables the vertebrate retina to grow with unchanged shape and keep its optimal function. Altogether, using a highly quantitative approach to development, 3D tissue-wide analyses and a combination of experiments and theory, we contributed to our understanding of the complex question of shape maintenance during growth. We think that more of such 3D studies and a more quantitative and interdisciplinary approach is necessary for developmental biology studies, whenever possible. This kind of investigation can allow us to characterize development in detail, improve rebuilding tissues in synthetic and organoid systems and, most importantly, fully understand the complex in vivo development that builds the world around us.
These findings are described in the article entitled A non-cell-autonomous actin redistribution enables isotropic retinal growth, recently published in the journal PLOS Biology. This work was conducted by Marija Matejčić and Caren Norden from the Max Planck Institute of Molecular Cell Biology and Genetics, and Guillaume Salbreux from The Francis Crick Institute.