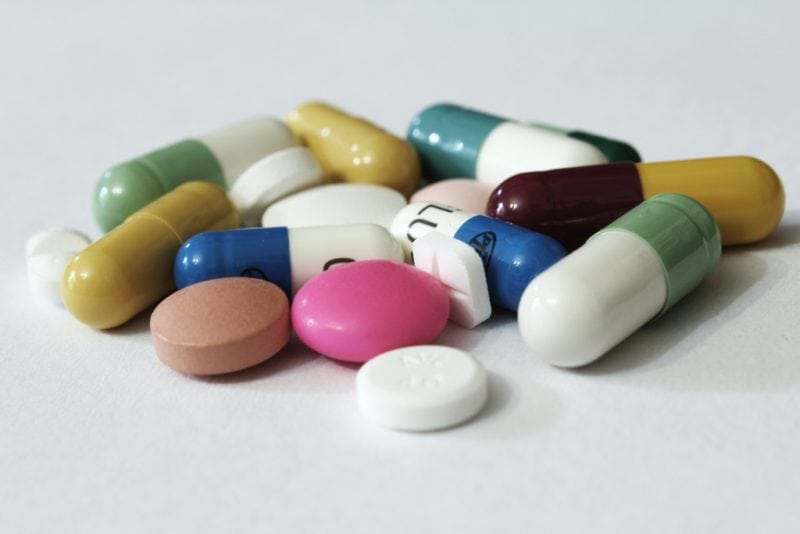
During the past decade, huge progress has been made in the field of drug discovery research. Several approaches have been explored to provide patients with treatments of the highest safety, with a high efficacy and minimum side effects. Different routes of administration are used to limit systemic exposure, and drugs are being (re)formulated to obtain optimal pharmacokinetics. The packaging of small molecules, proteins, and DNA or RNA has been explored to protect these from metabolic breakdown or from elimination by the immune system, and to obtain highly targeted, organ-specific or subcellular delivery.
When looking into the early stages of drug design, a drug’s molecular structure is optimized as much as possible, often taking multiple years, to obtain a very high selectivity for the target tissue and specific drug target involved. But even then, when the drug molecule reaches its target cell, we are still not where we want to be in terms of the therapeutic effect. The ultimate goal is to be able to describe a structure-activity relationship, which eventually links the molecular structure to a desired therapeutic effect.
One of the most intensively studied drug targets is the family of G protein-coupled receptors (GPCRs), targeted by approximately 35% of approved drugs available on the market today. These seven-transmembrane-spanning proteins are highly expressed on the cell surface in the human body and are activated by different endogenous ligands such as hormones, neurotransmitters, light, and odors. Because of their wide distribution and diversity, they represent ideal drug targets but at the same time, this implies that a very specific activation is required to avoid off-target pharmacology and thus side effects.
The binding of an endogenous or drug-ligand activates the GPCR and induces a conformational rearrangement in the transmembrane domains, which is transferred to the intracellular, cytoplasmic loops of the protein, there providing an activated site for the coupling with the G-protein. In such a way, GPCR activation by a ligand has been described for many years as a lock-key mechanism by molecular scientists.
Once the GPCR is “unlocked” by its ligand, the G-protein can couple and initiate further downstream signaling to so-called “second messenger” molecules. In this way, a message is conveyed from the cell surface to the inside of the cell, culminating in an impact on protein expression, cell metabolism, and/or cell behavior, eventually leading to a desired (therapeutic) effect. However, while this may seem relatively simple and straightforward, the reality turns out to have many levels of delicate complexity.
In more recent years, molecular cell biologists have further elucidated the intracellular coupling mechanism of GPCRs; this seems to not only involve G protein coupling but also signaling to other adaptor proteins. In this way, a single drug molecule can bind to and activate the GPCR, thereby initiating signaling to a diverse set of proteins, of which some will be responsible for the therapeutic effect(s), whereas others might produce side effects. This phenomenon is referred to as biased signaling of a ligand. This also means that we are entering a new chapter in drug discovery, in which newly synthesized drug molecules should be optimized to not only bind to a specific GPCR but should also induce coupling to a selective group of downstream GPCR adaptor proteins that will provide the desired effects, with minimal side effects.
One of the most studied signaling proteins, besides the G proteins, is the family of arrestin adaptor proteins. After ligand binding and GPCR activation, arrestins are recruited to the GPCR to limit downstream signaling. As the word implies, they “arrest” further signaling to keep the cell from going “into overdrive.” This phenomenon is referred to as “GPCR desensitization” and protects against sustained GPCR activation; for example, when having sustained high drug levels with chronic drug therapies. Besides, because of this desensitization, the stimulation of the GPCR and eventually the therapeutic effect will be reached more difficult, which might lead to drug tolerance. Arrestins have also proven to be responsible for side effects, as they can also initiate further downstream cell signaling.
Although it is still early days, some GPCR systems have been described for which biased signaling could provide an explanation for a desired therapeutic effect versus the profound existence of side effects. Examples of this are analgesic drugs of the opioid family such as morphine, working on opioid GPCRs, anti-Parkinson drugs working on dopaminergic receptors, and antihypertensives working on the vasopressin receptor family. With opioid receptors, G protein coupling is responsible for the desired analgesic effect, while arrestin coupling introduces the side effects such as constipation, sedation, and even tolerance. Very first new biased drug candidates are being explored for these GPCR systems.
At the Laboratory of Toxicology at Ghent University, Belgium, the coupling of different GPCR families with arrestins is studied. The lab developed a cellular bio-assay, in which the GPCR and arrestin molecule are linked to two parts of a bioluminescent enzyme molecule, respectively. Once a ligand is added to the cells, it binds to the GPCR and arrestin is recruited, upon which the activity of the enzyme is restored, producing light following the addition of a cell-permeable substrate.
This bio-assay has been used to test ligand activity at a GPCR belonging to the purinergic GPCR family, for which drug candidates are arising for treatment of rheumatoid arthritis and psoriasis, as well as certain types of cancer. Until now, the ligands of interest were mainly tested on their signaling towards the G-protein pathway. Therefore, additional testing on the arrestin pathway might be of relevance in the future, when beneficial effects versus side effects are explored. By modifying the GPCR in its intracellular loops, the Ghent researchers explored which part of this GPCR might be responsible for arrestin coupling so that a clear structure-activity relationship might be revealed for newly synthesized compounds.
The Ghent University lab has also used the arrestin-based bio-assay to introduce a quite novel concept in the forensic toxicological world, where it can be applied for screening of biofluids for the presence of synthetic cannabinoids and (synthetic) opioids. Conventional drug screening procedures include analytical procedures, mainly relying on liquid chromatography mass-spectrometry-based techniques, and immuno-assays, relying on recognition of a molecule by an antibody. In these cases, the exact molecular structure should be more or less known. However, drug labs are trying to evade legislation and drug screening by modifying existing drugs with minor structural changes, which has led to an emergence of designer drugs (“new psychoactive substances”). In the bio-assay, these synthetic drugs (e.g. synthetic cannabinoids) will still bind to their receptor (e.g. the cannabinoid receptor) and can hence be detected with high sensitivity.
These findings are described in the article entitled Molecular dissection of the human A3 adenosine receptor coupling with β-arrestin2, recently published in the journal Biochemical Pharmacology. This work was conducted by Jolien Storme, Annelies Cannaert, Kathleen Van Craenenbroeck, and Christophe P. Stove from Ghent University.