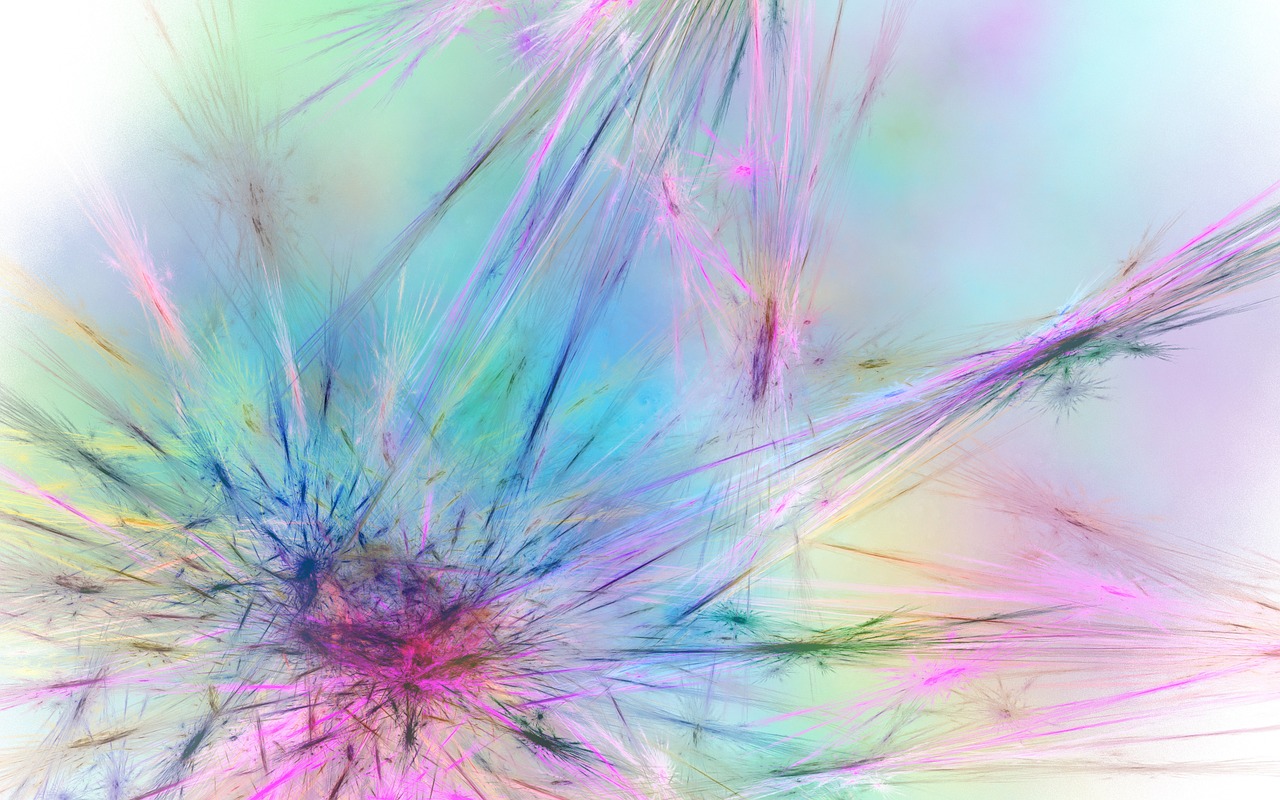
New research shows how computer-based math models can communicate with neurons to study neurodegenerative diseases.
Brain diseases such as epilepsy and neurodegeneration (e.g., Alzheimer’s disease, Parkinson’s disease, and others) affect millions of people all over the world. Scientists at the University of California Los Angeles are seeking ways to better understand what goes wrong in the brain cells during disease development. Even more fascinating is the fact that researchers are able to create mathematical models to manipulate sick brain cells to learn more about them.
Using a technique known as dynamic-clamp, Dr. Sharmila Venugopal, a faculty member in the Department of Integrative Biology and Physiology, and her colleagues interfaced neurons in live brain slices with computer-based mathematical models of ion channel physiology to investigate how disease can alter neurophysiology.
Normally, experimentalists use pharmacological or genetic perturbations to study how ion channel proteins function in neurons, heart cells, muscle cells, and other cell types.
“But these approaches are often associated with side effects of the drug or the genetic manipulation used,” notes Dr. Venugopal.
In their study, soon to be published in the Journal of Neuroscience, they used realistic math models of ion channel physiology to rescue normal electrical activity patterns in neurons in a transgenic mouse model for the neurodegenerative disease Amyotrophic Lateral Sclerosis, or ALS. Their experiments consisted of a real-time bio-hybrid interface between the computer-based math model and real neurons in brain slices.
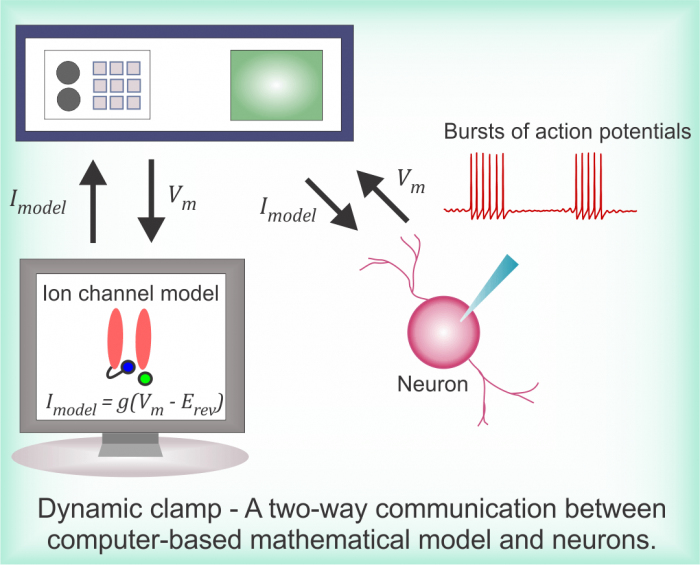
Image courtesy Dr. Sharmila Venugopal
They first established a closed-loop connection between the model and the neuron.
“It is like a hand-shake signal on the internet between a client and a server, but this communication is always two-way,” explains Dr. Venugopal, senior author of the study.
Using this approach, the team first examined how sodium channels work in sensory neurons in their recently-published study in PLOS Computational Biology. They were able to regenerate complex bursts of electrical signals known as action potentials driven exclusively by the model sodium currents after complete experimental blockade of sodium channels using a drug. They subsequently used this approach to restore deficits in sodium currents in ALS which also corrected the irregular patterns of action potentials seen during disease development. Identifying ways to restore normal activity patterns as suggested by their model-in-the-loop could help devise biomarkers and design effective interventional strategies.
Dr. Venugopal is an electrical engineer-turned-neuroscientist. She develops mathematical models to explain how diseases such as neurodegeneration and spinal cord injury alter normal physiology of the brain.
“Our brain is a very complex machine, and understanding its complexity requires many disciplines to come together, including mathematics and computer simulations. Math models are necessary because physiological variables are often experimentally intractable. Moreover, diseases are elusive, and models can help make important predictions to guide valuable new experiments,” she notes.
The study’s first author is Dr. Soju Seki, a postdoctoral scholar and Dr. Scott Chandler, professor of neuroscience, is the co-corresponding author. Dynamic-clamp experiments were conducted in collaboration with Dr. Riccardo Olcese Lab in the David Geffen School of Medicine at UCLA with contributions from Dr. Antonios Pantazis (co-author). The study was funded by the National Institute for Neurological Diseases and Stroke.