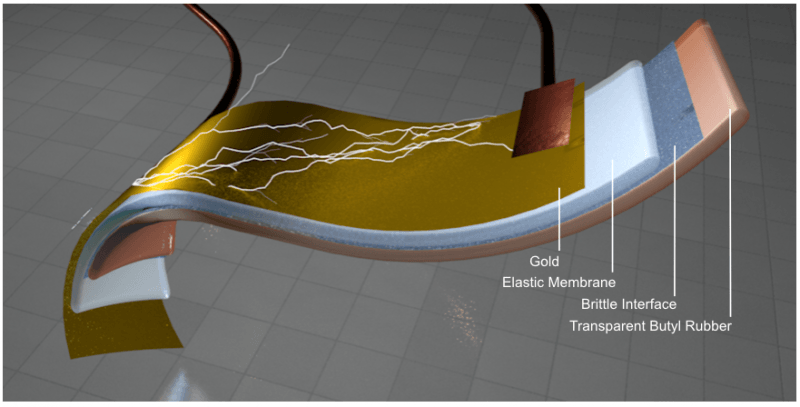
The future of electronics will include soft, stretchable devices that can conform to the human body for an entirely new and intimate human-computer interaction. The development of these new wearables uses an elastomeric substrate as a “base” for functional electronic components – devices and wires – that move with the elastomer to provide electrical function during bending, stretching, and twisting.
The elastomeric substrate is a key element in stretchable electronics: It must be soft enough to interact with humans, and at the same time mechanically stabilize on-board devices and protect sensitive device components from environmental conditions that can cause corrosion or degradation. It’s a tall order for a single elastomer.
Researchers at the University of Windsor, Canada recently reported a new approach to solving this problem. By fusing two different elastomers together to form a layered structure, the research team transformed the elastomeric substrate into a multifunctional element that combines the different properties of the two elastomers into a single robust structure, providing functionality that a single elastomer alone cannot deliver. The researchers demonstrated the idea using a “molecular glue” to adhere a thin membrane of a silicone rubber onto the surface of a transparent butyl rubber substrate, merging the robust surface chemistry of the silicone rubber with the intrinsically low gas- and moisture-barrier properties of butyl rubber. The resulting layered elastomer can bind to a layer of silver or copper deposited on the silicone rubber surface, and at the same time, protect these sensitive metals from atmospheric corrosion.
The research team named the new layered elastomers Membrane-INterface-Elastomer (MINE) structures to highlight the importance of the interface between the two elastomer layers, which contributed to the most intriguing result of this study. This interface, composed of brittle silica and molecular glue and buried tens of microns below the membrane surface, changes the mechanical behavior of metal films coated on the membrane surface above.
Stretching metal-coated MINE structures induces a uniquely favorable cracking pattern to form in the metal film through stress transfer, enabling it to remain remarkably electrically conductive to high elongations. MINE structures are thus more than simply the sum of two elastomers. The synergy exhibited by the elastomers and the interface between them presents a new approach to modifying the mechanical and electrical behavior of partner material coatings for a potentially broad range of applications. As a start, the research team demonstrated that metal films deposited on silicone rubber/butyl rubber MINE structures can be used as electrodes in large-area light-emitting that are intrinsically stretchable. These devices emit light during stretching to 1.5x the original device length, and function in high humidity or even underwater due to the moisture barrier protection afforded by the butyl rubber.
The entirely new design approach that MINE structures bring to stretchable electronics may lead to exciting new applications unattainable using a single elastomer substrate alone. The ability to combine favorable surface properties of the membrane with useful bulk properties of softness, strength, wettability, or tackiness, opens the way to stretchable and wearable devices with properties tailor-made to suit these applications. Furthermore, engineering the properties of the brittle interface of MINE composites to exploit stress transfer to an overlying partner material can lead to future “smart” MINE structures in which desired mechanical properties can be programmed into the brittle interface.
The findings are described in the article entitled Membrane-Interface-Elastomer (MINE) Structures for Stretchable Electronics, recently published in the journal Chem. This work was conducted by co-authors Akhil Vohra, Kory Schlingman, R. Stephen Carmichael, and Tricia Breen Carmichael from the University of Windsor.