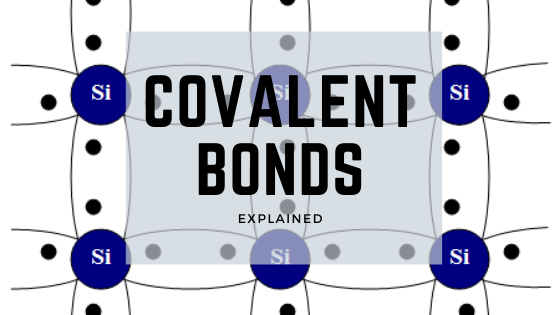
A covalent bond is a type of chemical bond characterized by the joint sharing of electron pairs between atoms. Covalent bonds form when atoms share valence electrons with other atoms to achieve a full shell of outer electrons. Covalent bonds differ from ionic bonds, which involve the removal or addition of electrons, and metallic bonds, which involve a non-localized sharing of electrons. Compounds that are formed from covalent bonded atoms are called covalent compounds.
Generally, covalent bonds form between non-metal elements that do not differ greatly in electronegativities. Covalent bonds tend to be weaker than ionic or metallic bonds, so they require less energy to break. As a consequence of these relatively weaker bonds, most covalent compounds are gases at standard temperatures and pressure and have low boiling and evaporation temperatures. Typically, with covalent bonds, elements will share electron pairs until each atom in the compound has 8 valence electrons, except for hydrogen (H) which only needs 2 valence electrons to fill its outer shell.
“Molecular chemistry, the chemistry of the covalent bond, is concerned with uncovering and mastering the rules that govern the structures, properties and transformations of molecular species.” — Jean-Marie Lehn
A simple example of a covalent bond is in a diatomic molecule of hydrogen (H₂) Hydrogen by itself has one valence electron. Hydrogen has a full shell whenever it has 2 electrons. So, a single hydrogen atom will share its single valence electron with another hydrogen atom, and vice versa. The result is that both hydrogen atoms now have a full outer shell, and the molecule has a stable electron configuration.
In some cases, atoms will share more than just two electrons. Atoms can form double and triple bonds, where they share 4 and 6 electrons, respectively. Atoms will form double and triple bonds if every electron pair is shared, but some atoms still need more electrons to fill their outer shells.
Types Of Covalent Bonds
Covalent bonds can be divided into two main categories, polar covalent and non-polar covalent. Whether or not two atoms will form a polar or non-polar covalent bond depends on those atoms’ respective electronegativities.
The electronegativity of an element can be thought of as a measure of how “hungry” an element is for electrons. The more electronegative an element, the more “hungry” it is for electrons, and the more it pulls on electrons. Elements to the right of the periodic table tend to be more electronegative in virtue of the size of their positively charged nucleus. The larger positive nuclei found in the elements to the right of the periodic table tend to exert a greater pull on electrons. Fluorine (F) is the most electronegative element and is assigned an EN value of 4. All other EN values are calculated with respect to fluorine.
Covalent bonds are generally defined as bonds that occur between elements whose difference in electronegativities is ΔEN ≤ 1.8. When the EN difference between elements is greater than 1.8, the elements are said to engage in ionic bonding instead of covalent bonding.
“Moreover, the abundance of chemical compounds and their importance in daily life hindered the chemist from investigating the question, in what does the individuality of the atoms of different elements consist.” — Johannes Stark
Polar Covalent Bonds
When the EN difference between elements is between 0.4 and 1.8, the formed covalent bonds are called polar. In polar bonds, the more electronegative element exerts a greater pull on the shared electrons than the less electronegative element. The result is that shared electrons are pulled more towards one atom. This net-difference in electric charge across the bond makes it so the covalent bond has a partially charged negative end and a partially charged positive end.
The polarity of a compound explains a number of its physical structure, such as its 3-dimensional geometry, intermolecular interactions, and phase behavior. For example, water is a covalent polar compound. The polarity of the covalent bonds in water explains its solvent capabilities, high boiling point, high specific heat capacity, surface tension, and intermolecular behavior.
Non-polar Covalent Bonds
When two atoms that have nearly identical EN values bond, they form non-polar covalent bonds. In non-polar covalent bonds, electrons are shared equally between the two atoms, so there is no net difference in charge across the bond. Bonds formed between atoms of the same element are considered completely non-polar, as the two atoms have identical EN values and so no difference.
For instance, chlorine gas is composed of diatomic chlorine molecules (Cl2). Each atom of chlorine has 7 valence electrons, distributed into 3 pairs and a single lone electron. Each chlorine atom will share its lone electron with the other, giving each chlorine atoms 4 electron pairs and so 8 total valence electrons. Because identical elements have identical EN values, both chlorine atom exerts an equal pull on the shared electrons and cancel each other out. Non-polar bonds are completely electrically neutral.
For a compound to be non-polar, it does not have to be composed out of non-polar covalent bonds. Consider carbon tetrachloride (CCl4). Carbon tetrachloride is formed by a carbon atom forming 4 covalent bonds with 4 chlorine atoms. The ΔEN between carbon and chlorine is 0.5, so C-Cl bonds are polar. However, carbon tetrachloride is a non-polar molecule. The reason why is that the symmetric tetrahedral geometric structure of carbon tetrachloride makes it so each polar bond is canceled out by another.
How Do Atoms Share Their Electrons?
It is one thing to describe a covalent bond as the sharing of electron pairs; it is another thing entirely to explain how atoms share their electrons. That is a question for molecular orbital theory.
Common depictions of electrons visualize them as tiny particles that orbit around a central nucleus, analogous to the planets in our solar system. Lewis structures, for instance, represent electrons as little dots surrounding a central atom, and covalent bonding is represented as two atoms sharing a pair of these dots. These kinds of models are not accurate representations though. Electrons are not tiny individual particles that whiz around atoms like planets in a circular orbit. Electrons have a dual particle-wave nature, and their bonding behavior can only be explained by the wave-like properties of electrons.
Since electrons are not particles, they do not have a definite position at a specific point. Instead, like a wave, they exist spread out in a region of space. This region of space is called an orbital. In some ways, one can think of an orbital as a region of space around an atom in which there is a probability of finding an electron.
“Nothing exists except atoms and empty space; everything else is opinion.” — Democritus
Atoms form covalent bonds by overlapping their electron orbitals. When two atomic orbitals come close, they combine and the electrons begin to inhabit a shared orbital space. In this shared orbital space, there is an increased electron density. The electron density in this shared orbital space is the “chemical glue” that holds the atoms together There are many kinds of electron orbitals, so there are many kinds of orbital overlap. The exact character of orbital overlap determines the bond’s strength, angle, length, and elasticity.
For example, a diatomic hydrogen molecule (H₂) has the simplest and most stable type of covalent bond; a sigma bond (represented as σ-bond). Sigma bonds form as the result of the direct overlap of two s orbitals. s orbitals have a spherical shape, so the shared orbital space is like the region that forms from the overlap of two spheres. Pi-bonds (π) bonds, in contrast, form due to the lateral overlap of 2 p orbitals. When two orbitals combine the resulting orbital is called a hybrid orbital.
Think of the concept this way: imagine you drop a rock in a body of water and waves ripple out. You then drop a rock at a different point which creates another pattern of waves. When the ripples meet, the waves will combine in various ways to form different patterns. This is kind of like what is going on in electron orbital overlap. Electrons are fundamentally wave-like, so when you bring two electrons close together, the waves will “combine” to form a distinct stable wave pattern. The properties of a covalent bond are determined by the exact shape of the orbitals, and how those waves combine with each other. The orbitals will combine and take on a shape that minimizes the potential energy between the atoms.
Orbital theory provides a more accurate description of electrons and an explanation for why electrons behave in the way they do when forming shared pairs. Orbital theory can also be used to predict the character of novel chemical bonds. It also explains why some compounds, like sulfur hexafluoride (SF6) violate the octet rule on their central atom.
To recap, covalent bonds are chemical bonds characterized by the sharing of electron pairs. A covalent bond is formed when two atoms share electrons in order to gain a complete outer shell of electrons. There are two main kinds of covalent bonds, polar and non-polar, that are distinguished based on how equally electrons are shared across the bond. Atoms share electrons by overlapping electron orbitals into a shared orbital space. The increased electron density in the shared orbital space anchors the two atoms together.