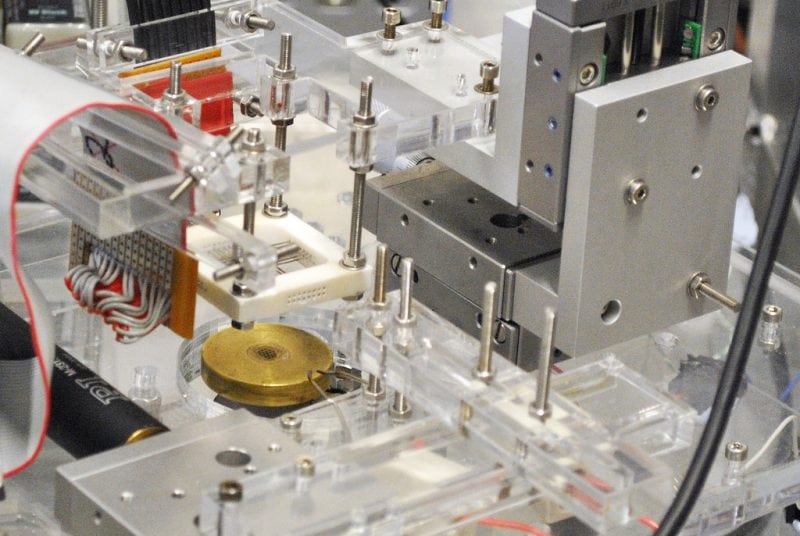
Anion exchange membrane (AEM) is like plastic wrap in the kitchen, but it consists of a polymer backbone and positively charged functional groups, which can exchange anions. The use of AEM is growing quickly in electrochemical energy conversion devices (alkaline fuel cells) and energy storage systems (e.g. flow batteries).
As its core component, AEM must have good hydroxide conductivity, limited water uptake, and decent chemical stability. The conductivity of AEM is often low, because of the lower mobility of larger ions — for example, Cl– and OH–, compared to H+, which is much smaller in size. A straightforward method to increase the conductivity of AEMs is to incorporate more positively-charged groups, i.e. increasing the ion exchange capacity. However, the mechanical integrity of the AEMs is then compromised because of severe water swelling.
Covalent crosslinking can reduce AEM swelling and minimize the chance of hydroxide ion attack on either the functional groups or the polymer backbone. Traditional strategies to crosslink AEMs include, “click” chemistry, Menshutkin reaction, olefin metathesis, ring-opening metathesis polymerization, and thermal crosslinking.
Nevertheless, crosslinking in these processes lacks proper control, since crosslinking happens at the same time the membrane is drying. Besides, the solution always turns to gel before the membrane could be cast. A high temperature is sometimes required.
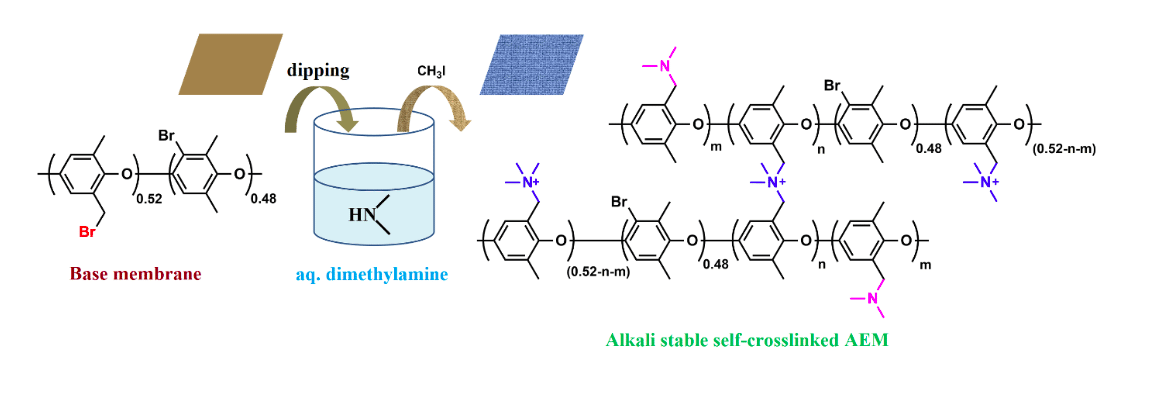
Figure 1: Fabrication of self-crosslinked membrane by immersing halo-alkylated polymer membrane in dimethylamine (aq.) and methylation. (Republished with permission from Elsevier)
To reduce the complexity in making a cross-linked AEM, we report a straightforward procedure to prepare a highly crosslinked, transparent, and flexible AEM by simply immersing a halo-alkylated membrane in aq. dimethylamine solution at room temperature (see figure 1). When a fresh membrane is immersed in aq. dimethylamine solution, it gets cross-linked immediately and the tertiary amine group can be further quaternized to control ion exchange group content of the membrane.
The resulting membranes have improved tensile strength (up to 19.8 MPa) and crosslinking significantly reduces the membrane water uptake and linear swelling ratio, resulting in improved dimensional stability. For the non-crosslinked membrane, water uptake approaches 126 wt% at room temperature, whereas crosslinked membranes show a 3~6-fold decrease in water uptake (20-42 wt%), which is slightly changed as temperature increases.
The self-crosslinked membranes show a hydroxide conductivity of 26 mS cm-1 at 25°C. Moreover, hydroxide conductivity of the crosslinked membrane increases significantly over a temperature increase, while the swelling ratio increases very slowly. The membrane is also chemically stable under highly basic conditions. We found no decline in hydroxide conductivity after immersing the membrane in 1 M KOH at 60°C for 360h. In contrast, the control, a non-crosslinked QPPO membrane, lost ∼60% of the original conductivity. We attribute such improvement to the restricted hydroxide ion attack and minimized Hofmann elimination reaction (no β-hydrogen present). A H2/O2 fuel cell assembled with this membrane exhibited a peak power density of 31 mW cm-2 at 60 oC.
The work is significant as it introduces an easy way to prepare highly self-crosslinked AEM, which has high conductivity and good chemical stability. The crosslinking procedure developed in this paper is very promising and can be potentially applied to a series of halo-alkylated polymer membranes, which would enable the production of novel AEM and its application in electrochemical devices, to generate or to store electricity.
These findings are described in the article entitled Anion exchange membrane crosslinked in the easiest way stands out for fuel cells, recently published in the Journal of Power Sources. This work was conducted by Md. Masem Hossain, Liang Wu, Xian Liang, Zhengjin Yang, Jianqiu Hou and Tongwen Xu from the University of Science and Technology of China.