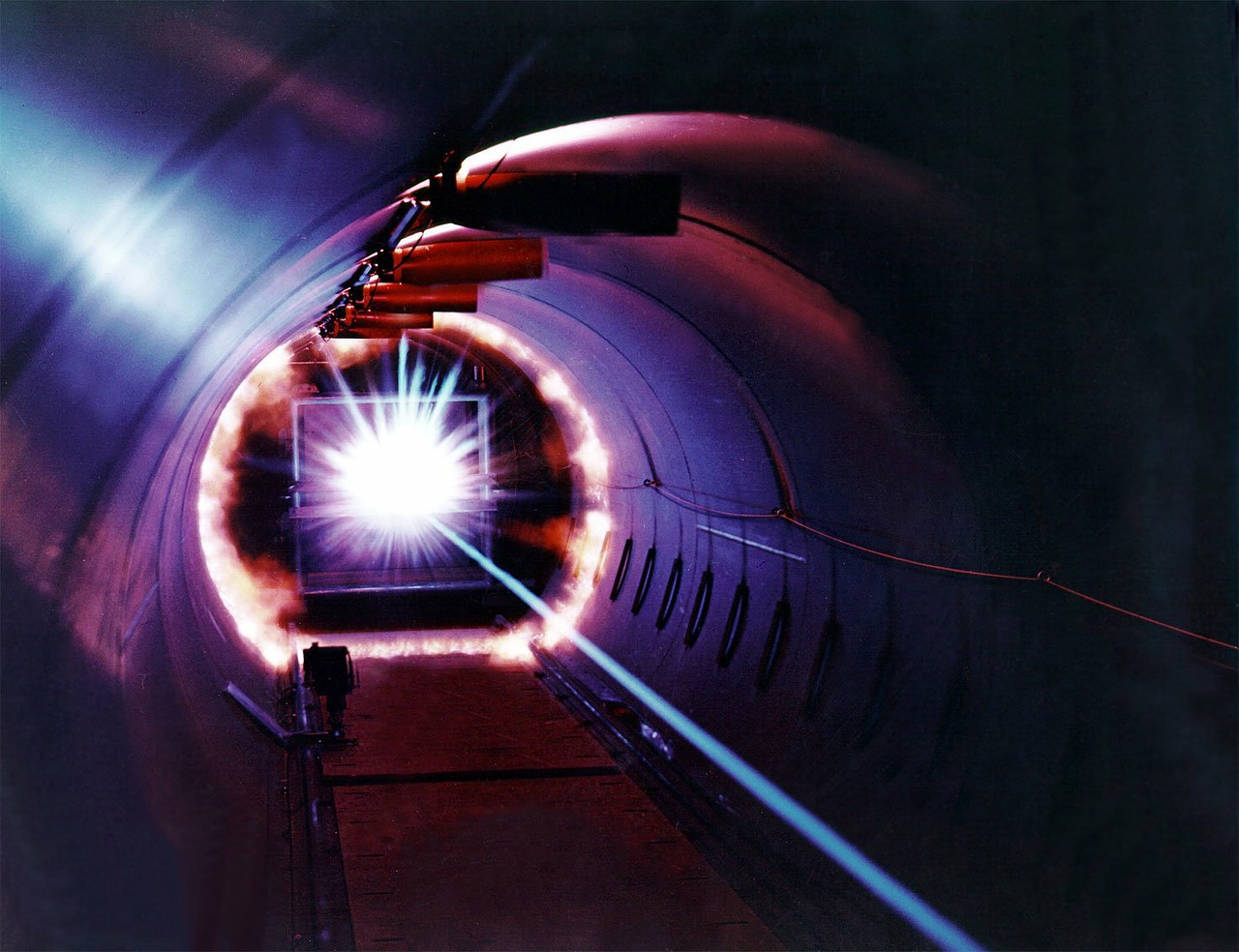
Light is made of electromagnetic waves, which are composed of a time-varying electric field and time-varying magnetic field. The time-varying electric field can be described as the oscillation behavior of the light waves. The electric field of a coherent light has its own spatial-temporal oscillation behavior, which is called polarization state of light.
Dependent upon oscillation behavior, the polarization state of light can be classified into elliptically, circularly, and linearly polarized lights. The polarization state of the light wave is highly dependent upon the amplitude and phase of the time-varying electric field. When light is obliquely incident on a smooth surface of a material, due to the phase and the amplitude changes of the time-varying electric field, therefore, the polarization state of the reflected light wave can change.
In general, this polarization state of the reflected light is elliptically polarized. Ellipsometry is a high-precision measurement technique for measuring material properties by analyzing the polarization state of the emergent light reflected from the material’s surface. In 1945, Alexandre Rothen designed an instrument that measured the thickness of the film on a metal substrate and designated the optical setup as an ellipsometer, which is based on the concept of ellipsometry [1]. The ellipsometer is a very useful apparatus to measure the optical properties including refractive index and thickness of a thin film layer (about several nanometers to several hundred nanometers). This measurement technique can also be applied to the semiconductor industry, as well as biomedical and material sciences.
The interferometric ellipsometer is an optical instrument that successfully integrates interferometry into the ellipsometry. The first interferometric ellipsometer was proposed by Hazebroek and Holscher [2]. Conventional interferometric ellipsometers are all based on an amplitude-division interferometer, such as a Michelson or a Mach–Zehnder interferometer [3-5]. The amplitude and phase of the reflected light can be extracted from the temporal interference fringes of the interferometer.
The conventional interferometric ellipsometers permit the measurement accuracy to be very high. However, with this result, it is also assumed that the optical system setup of the used interferometer is stable and the optical or photoelectrical properties of the used component in the optical system setup are ideal. In fact, if the used components in a conventional interferometric ellipsometer are not ideal, the imperfection of system components affects the measurement accuracy in conventional interferometric ellipsometers. Owing to the fact that amplitude-division interferometer is highly phase sensitive, the surrounding perturbations in the optical path contribute to the significant change of the interferometric fringe; therefore, phase noise is induced. In order to increase measurement accuracy, the system stability of the conventional interferometer should be maintained in the measurement process.
Improving measurement accuracy and making the system setup become robust are two important issues for developing an interferometric ellipsometer. Recently, our research group has proposed a new design of an interferometric ellipsometer that can have greater measurement accuracy but a simpler optical system setup. We designated this optical system setup as a simple heterodyne interferometric ellipsometer (Figure 1).
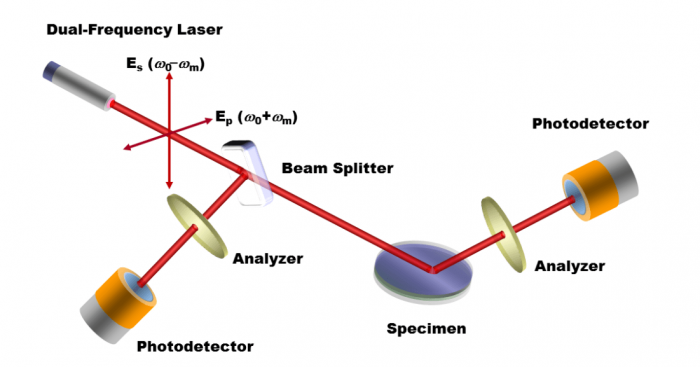
Figure 1. Optical Setup of the simple heterodyne interferometric ellipsometer. Figure courtesy Chih-Jen Yu.
Different from the conventional interferometric ellipsometer, the proposed interferometric ellipsometer utilizes a dual frequency laser [6] and a common path interferometer. The virtue of the common path interferometer can effectively reduce the surrounding perturbations since the reference beam and signal beam propagate the same optical path and hence the common phase noise can be eliminated simultaneously.
To improve the measurement accuracy, we carefully considered the system error of used components in the proposed optical setup. In this setup, we considered that the system error could come from the following error sources: the transmission and reflection properties of the used beam splitter surface changes the polarization state of the incident light, and the non-identical photoelectrical response of a couple of the photodetectors used in the optical system setup may affect the amplitude of the detected signal of the signal beam and reference beam.
To reduce the effect of these error sources, we reconsidered the imperfect properties of the used optical and photoelectrical components. While the tested specimen is not placed before, we measured the actual properties of these components as a calibrated parameter of a system calibration. Once these imperfect properties were measured, the measured error could be compensated and, hence, the measurement accuracy could be well improved. Additionally, similar to the conventional interferometric ellipsometer, the amplitude and phase can also be extracted from the interferometric fringes to suppress the noise induced by the optical setup and electronic instruments. The amplitude and phase are obtained by nonlinear curve fitting with the measured interference fringes.
With the help of this numerical analysis process, the effect of fluctuation in the interference fringe can help reduce the fluctuation of interferometric fringes.