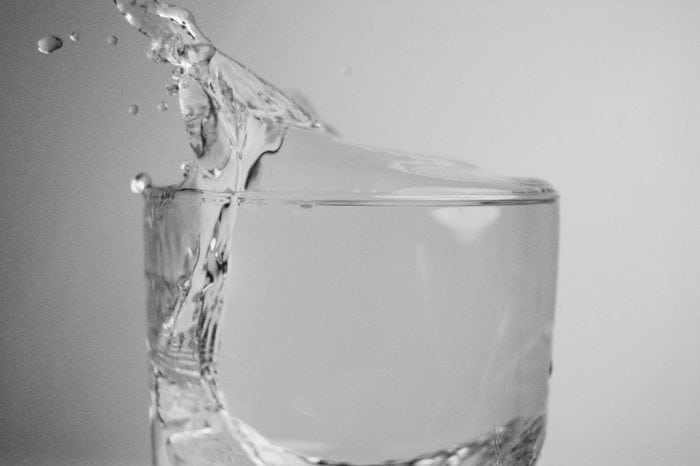
Paracelsus’ old adage “the dose makes the poison” is well exemplified by fluoride. Many people know that fluoride is commonly added to drinking water supplies to promote dental health and lower the risk of cavities. And while low levels of fluoride are indeed safe and effective, exposure to elevated fluoride levels can cause dental fluorosis and other serious health problems (“Water Fluoridation Controversy” even merits its own Wikipedia page [1]). As a result, water fluoridation is carefully monitored to ensure that fluoride levels are kept in the proper range. For example, New York City releases an annual “Drinking Water Supply and Quality Report” that includes data on measured fluoride levels — the most recent 2017 report describes fluoridation data taken for over 2,000 samples [2].
To increase the accuracy and efficiency of fluoride level monitoring, chemists have put a great deal of effort into developing methods of sensing fluoride in solution. In a recent study titled Fluoride Detection with a Redox-Active Naphthalene Diimide Metal–Organic Framework, published in the journal Polyhedron, our group has reported a new strategy for developing fluoride sensor devices [3].
What Makes a Good Sensor
Any sensor needs to do two things efficiently: interact selectively with the thing that you are trying to sense (known as the “analyte”); and, transmit data to the user about the presence or absence of that thing (known as “signal transduction”). Particularly useful are signal transduction mechanisms that give the user an easy readout. One of the most common and useful signal transduction mechanisms is color change, such as in commercially available kits for testing lead or chlorine in water or pH litmus paper. Another common readout is based on a change in electrical signal, such as in a typical blood glucose meter.
Metal—Organic Frameworks: A New Platform for Sensor Development
Over the past several decades, a new category of materials known as metal–organic frameworks (or MOFs) has gained significant attention for their promising applications in various technologies [4]. As a result of how they are made, two hallmark features of MOFs are (1) highly tailorable structure and chemical properties and (2) record high porosity and surface area. Both of these properties make MOFs rather ideal “designer” materials for chemical sensors: the controllable, high-surface-area structures can allow for sensitive and selective interactions with an analyte of interest (such as fluoride).
A significant challenge of MOF-based sensors has been signal transduction. In the early years of MOF chemistry, very few MOFs displayed easily observed responses such as a change in color or an electrical response [5]. Only recently have strategies been developed that have allowed for a new generation of MOF-based sensor devices [6]. From a chemical perspective, a key feature of many MOFs that exhibit desirable sensing behavior is redox activity; that is, the material can gain or lose electrons and thereby change its properties [7]. The development of new redox-active MOFs in recent years is enabling a wave of advances in sensing technologies.
A MOF-Based Fluoride Sensor
Our work makes use of chemistry in which organic molecules called naphthalene diimides (or NDIs) can serve as color-change sensors for fluoride in solution. It is known that, in the presence of fluoride, some NDIs can undergo reduction (gain of an electron) and thereby change color dramatically [8]. This color-change response is selective for fluoride, and no response is typically observed for a variety of other common ions found in water. A drawback, however, is that most of this work has required the NDI molecules to be dissolved in solution along with the fluoride ions — more desirable would be simple, solid-state sensor devices, equivalent to commonly used paper test strips for other ions in water.
To address these limitations, we used a porous MOF material that incorporates the reactive NDI molecules into its structure [9]. We envisioned that the high porosity would still allow the NDIs to be reduced in the presence of fluoride, even though they were not dissolved in solution. Gratifyingly, we found that the NDI-based MOF exhibits a dramatic color change from orange to black in the presence of fluoride-containing solutions, but not in the presence of other common ions. Detailed characterization of the MOF before and after exposure to fluoride confirmed that the color change is due to the reduction of the NDI molecules contained within the MOF. The incorporation of NDIs into a solid MOF allowed us to fabricate simple “dip strip” test papers that change color when dipped into fluoride-containing solutions. Furthermore, we found that the color change is reversible, which means that this technology could be used to make reusable fluoride sensor devices.
Challenges and Future Directions
Overall, we believe that incorporation of reactive, fluoride-sensing molecules into porous MOF materials represents a general strategy for accessing improved fluoride sensor technologies. Our recent study is a promising proof-of-concept demonstration, but more work will be needed to develop truly practical sensors. For example, many MOFs (including the one used in our study) display very limited stability in water. This is an obvious problem for applications such as monitoring fluoride content in water. Future work will need to target MOFs that have more robust stability in aqueous fluoride solutions. Additionally, the amounts of fluoride used in our initial study are significantly higher than would be found in fluoridated drinking water (typically around 0.7 mg/L, as recommended by the US Department of Health and Human Services) [10]. New and improved MOF-based fluoride sensors will need to be optimized for increased sensitivity towards fluoride in order to achieve real-world impact.
These findings are described in the article entitled Fluoride detection with a redox-active naphthalene diimide metal–organic framework, recently published in the journal Polyhedron. This work was conducted by Hanna C. Wentz and Michael G. Campbell from Barnard College.
References
- https://en.wikipedia.org/wiki/Water_fluoridation_controversy
- http://www.nyc.gov/html/dep/html/drinking_water/wsstate.shtml
- https://doi.org/10.1016/j.poly.2018.08.005
- https://pubs.acs.org/doi/10.1021/cr300014x
- https://pubs.acs.org/doi/10.1021/cr200324t
- https://doi.org/10.3390/s17051108
- https://doi.org/10.1039/C6CC00805D
- https://pubs.acs.org/doi/abs/10.1021/ja107382x
- https://doi.org/10.1016/j.chempr.2016.06.013
- https://www.cdc.gov/fluoridation/guidelines/index.htm