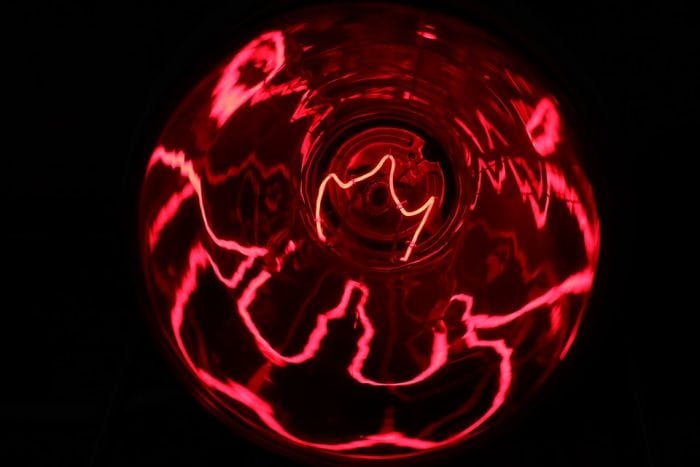
Thermoelectric emerged as the most promising technology to collect thermal energy, which is greatly abundant and universally existing but easily wasted in our living environment. Thermoelectric devices, like a heating engine without any bulky and moving parts, make it possible to recover heat in an environment quietly, reliably, and on a large scale. The thermoelectricity can be generated by the temperature gradient across a specific material/device based on the thermoelectric effect.
However, how to harvest thermal energy by using thermoelectrics when the environmental temperature is spatially uniform without any gradients is still a crucial issue and needs to be conquered. The key challenge is to create a significant temperature difference (ΔT) across the device to drive thermoelectric generators.
In addition to the direct heat source, a light source (such as the infrared light) can also provide thermal energy through the photothermal effect. Based on the photothermal effect and Seebeck effect, photo-thermoelectric generators have been rapidly developed for converting photo-energy into electricity without a spatial temperature gradient in the environment.
To generate a necessary ΔT, the conventional approach is to utilize various bulky components such as vacuum enclosures, condenser lenses, and heat sinks. Nevertheless, these extra modules will not only increase the weight and size of thermoelectric generators but also are unfavorable for the flexibility of the whole devices which is of great importance for wearable electronics. Motivated by the need for a practical application, this paper introduces a flexible photo-thermoelectric nanogenerator (PTENG) by integrating a MoS2/PU photothermal layer with a Te/PEDOT film-based thermoelectric device.
The photo-thermoelectric nanogenerators (PTENG) consisted of one flexible PET substrate, one layer of Te/PEDOT nanocomposites, which served as the thermoelectric materials, a pair of Ag electrodes, and a layer of MoS2/PU film located on top of one electrode, acting as a photothermal agent. The surface morphology and structure properties of the as-prepared samples were characterized by FESEM and Raman spectroscopy, respectively.
To examine the photothermal performance of the fabricated MoS2/PU film, an IR laser was used to illuminate the surface of photothermal films with different weight percentages of MoS2 for 200 s. Meanwhile, an IR camera was employed to record the sample temperature in situ. The results show that MoS2 is an excellent photothermal agent with high absorption efficiency of IR light.
A metallographic microscope was used to investigate the surface morphology of the MoS2/PU films. MoS2 nanoclusters distribute increasingly dense and uniform in the photothermal layer with the increasing weight percentage of MoS2 corresponding to the enhanced photothermal performance. Breakages and cracks will occur and appear in the film when the content comes to 3 wt%. This drawback is unfavorable for the transfer process. Therefore, based on the above discussion, the photothermal film with MoS2 content of 2 wt% was chosen to fabricate the PTENG.
As for the TENG part, Te nanowires were selected as the thermoelectric materials and aqueous PEDOT:PSS solution was used for the Te/PEDOT film formation to improve the electrical conductivity of the thermoelectric layer. A hotplate was applied as a heat source to generate a ΔT between two electrodes to examine the output performance of the TENG. The Seebeck coefficient is estimated to be 42 μV/K. There is a nearly linear increasing trend of open circuit voltage with the increasing of temperature difference, which indicates its potential application in self-powered temperature sensors.
For the PTENG part, MoS2/PU film was transferred onto one of the electrodes in TENG. This assembled device exhibits excellent flexibility and deformability, which makes it shape-adaptive and thus applicable to even curved surfaces for wide practical applications. The result demonstrates that the higher the laser power was introduced, the larger voltage could be achieved. This reveals a strong dependency between laser-power and photothermal effect for MoS2/PU film. The curves of photo-thermoelectric characteristics are similar to that of the photothermal property, which indicates that the temperature difference between the two electrodes is the driving force for the electrical output and the primary working mechanism of PTENG is formed by coupling the photothermal effect and Seebeck effect. It is worth noting that the electrical output shows no obvious decay after 200 s illumination. Actually, the PTENG can stably generate steady electrical output under the illumination of IR laser for even 10 min.
To explore the practical application, an infrared lamp was used to illuminate the PTENG which was attached to a window, and the potential difference was generated. Also, the photo-thermoelectric output can be generated when the PTENGs were exposed to the outdoor sunlight.
This work is significant in practical application owing to the unique advantages of the flexible PTENG. First, the PTENG can stably generate electrical output for a long time (as long as 10min) without any bulky cooling components such as a vacuum enclosure and heat sink. As a result, the device is light-weight and compact-size for wearable electronics. Secondly, the photo-thermal layer utilized in the PTENG is based on a simple MoS2/PU film which does not require complicated design process such as superlattice structures. In addition, the MoS2/PU photothermal layer is non-toxic and biocompatible, which is essential for the wearable electronics. Consequently, considering the high flexibility of the device, the PTENG has a great application potential in photo-thermoelectric energy harvesting for wearable electronics.
These findings are described in the article entitled A flexible photo-thermoelectric nanogenerator based on MoS2/PU photothermal layer for infrared light harvesting, recently published in the journal Nano Energy. This work was conducted by Yannan Xie from Xiamen University and Zonghong Lin from National Tsing Hua University.