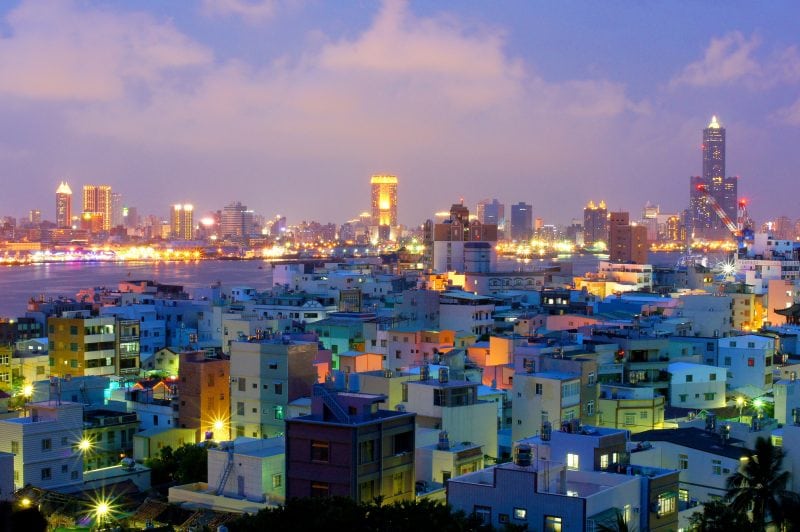
Multiple energy systems (MES) denote the integration of the generation, transmission, storage, and consumption of electricity, heat, cooling, and gas subsystems in modern energy systems. Driven by information and communication technology, the increasing coupling of different energy systems enables a smarter management of energy than operating each kind of energy system separately. For example, MES is able to improve the overall energy efficiency by synthetic cascade utilization of energy. It can also explore extra flexibility to accommodate more renewable energy integration.
China is the largest energy-consuming country in the world. Urban energy systems consume the most significant fraction of energy in China. Urban energy systems have high energy demand intensity, making energy efficiency of great concern. With the development of the electricity market reform and action plan of energy internet, the integrated energy service is open to public investment. Private companies are allowed to invest and be in charge of the energy supply of the newly-built urban area. An integrated planning of urban energy system planning is necessary to coordinate different forms of energy to achieve a higher efficiency and accommodate more renewable energy.
However, it is not an easy job to conduct such one-stop planning because of three challenges: 1) the electricity, heat, cold, and gas system belongs to different utility and there is no experience before to jointly design the whole energy system; 2) the physical fundamental models of electricity, heat, cold, and gas systems are distinct; 3) the coupling of different energy systems yields a much larger extra optimization space for planning the configuration, where there is no mature methodology to adopt. To fill these gaps, this study proposes a systematic and standardized modeling approach that facilitates the integrated planning of MES. The methodology is later used in the study of a typical urban energy system in northern China.
The concept of an energy hub (EH) is used in this study. An EH models an MES as a unit with multiple input and output ports, as shown in Fig. 1. The energy conversion, storage and distribution in MES should be modeled inner the EH. A standardized matrix modeling can be described based on graph theory, where the characteristics of energy converters/storage and their topology are expressed in matrix form. Fig. 2 shows the connections between the three energy converters (#1, #2, and #3) of a simple illustrative MES.
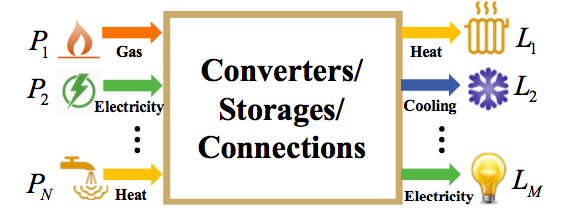
Fig. 1. Dual port “black box” model of an EH. (Republished with permission from Applied Energy)
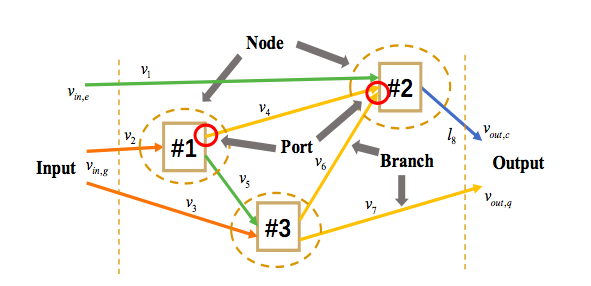
Fig. 2. Definitions of the basic elements of an EH in terms of graph theory (Republished with permission from Applied Energy)
Each energy converter in an EH can be treated as a node in a graph. Energy hubs can then be analyzed using graph theory if we map their physical components to graph theory concepts as follows:
- Each energy flow to or from a converter is represented by a branch. Branches, therefore, carry energy flows in any vector. (e.g. gas, electricity, heat, cooling)
- A node is the abstraction of an energy converter and also the abstraction of the branch endpoints. Inputs and outputs are treated as special nodes.
- Each node has one or more ports through which it exchanges energy with other nodes. Each converter has a fixed number of input and output ports. For example, a CHP unit has one input port for gas and two output ports for electricity and heat.
- A graph is a collection of nodes and branches.
- An EH is an oriented graph because each branch has a specified direction, i.e. from a source to a sink.
The standardized matrix modeling method provides a linear modeling solution for MES. It can also deal with the nonlinear energy conversion function in a piecewise linear way. More importantly, the proposed method uses graph theory to cast the topology and the characteristics of the energy converters into matrix form, which makes the analysis of MES be easily automated by computers. The method also provides a way to analytically analyze the inherent flexibility of the EH according to the degree of freedom of the linearized formulation.
Based on the automatic and standardized modeling of EH, an optimal EH configuration planning “starting from scratch” is proposed. The optimal EH configuration planning in this work address two challenges compared with traditional EH planning problems: 1) traditionally, part of the configuration of the EH and the types of energy converters have already been decided. In this study, there is no presumption about the configuration or the converters to be chosen for the EH; 2) most of the existing literature only optimizes the capacity of the converters. In this study, we optimize simultaneously the capacity of the converters and the configuration of the EH. Planning an EH “starting from scratch” means that no a priori assumptions are made about the choice of the energy converters and energy flow configuration.
Fig. 3 provides a general illustration of the proposed planning problem. Initially, the EH is empty. Various energy converters and storage devices with different capacities, efficiencies, and investment costs can be selected and connected to each other. The converters include CHP units, gas boilers, EB, heat pumps, transformers, absorption chillers, air conditioners, power to gas units, etc. The storage devices include heat storage and electricity storage. The demands for heat, cooling, and electricity can be forecasted and are used as boundary conditions of the proposed planning problem. The prices of the input energy sources, such as electricity and gas, are also known. Optimal configuration planning of an EH determines which energy converters should be selected and how they should be connected to minimize the overall cost, i.e. the sum of the investment and operating costs, for given energy demands and prices. The problem can be stated as follows:
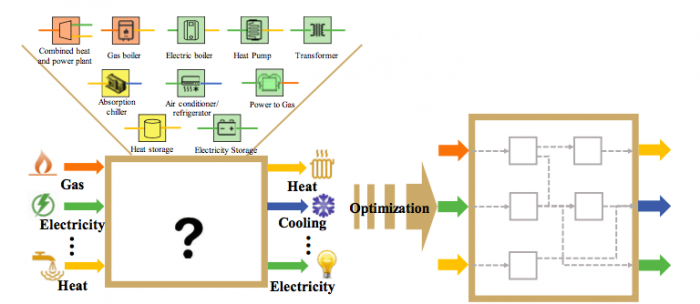
Fig. 3. Illustration of EH planning starting from scratch. (Republished with permission from Applied Energy)
We use the proposed method to study the optimal configuration of a typical urban energy system in northern China. The studied urban energy system covers the area of 6.0 square kilometers with the floor area of approximately 3.8 million square meters. The heat demand, cooling demand, and electricity load demand are shown in Fig. 4, such energy demand profile is representative in northern China.
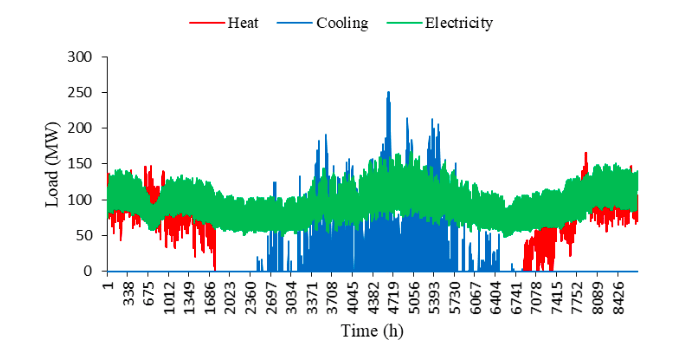
Fig. 4. Year-round heat, cooling and electricity demand of a typical urban energy system in Northern China. (Republished with permission from Applied Energy)
The optimization result of the MES configuration planning is presented in Fig. 5. As the optimization result shows, 60 MW combined heat and power (CHP), 30 MW electric heat pump (EHP), 40 MW compression electric refrigerator group (CERG), 80 MW water absorption refrigerator group (WARG), 360 MWh heat storage (HS), 135 MWh cooling storage (CS) and 46.5 MW photovoltaic (PV) system were chosen as the optimal planning scheme. The electricity demand is satisfied by the purchased electricity, CHP and roof PV system.
All roofs were suggested to install PV system because of their low operational cost and low investment cost in China today. Heat demand is satisfied by CHP and EHP. Cooling demand is satisfied by WARG and CERG. CHP and WARG were chosen to avoid purchasing a lot of electricity to meet the demand during peak and flat times. In addition, heat/cooling storage systems were chosen to reduce the heat/cooling cost by storing thermal/cooling power during valley times and discharge it during peak times.
The obtained MES configuration provides a basic blueprint for building an efficient urban energy system in China.
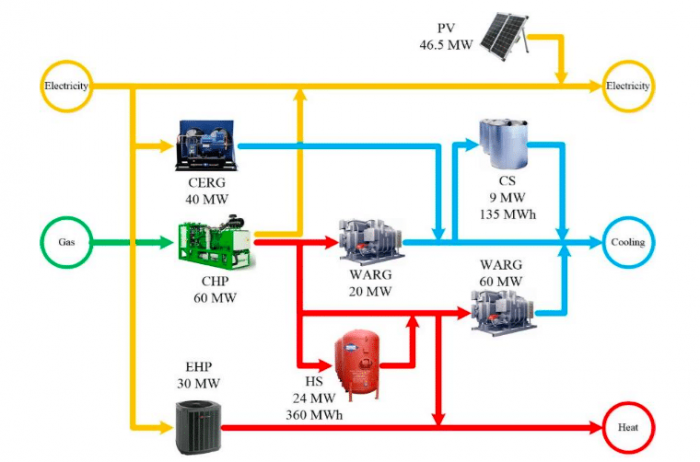
Fig. 5. Optimal configuration of the typical urban energy system in Northern China. (Republished with permission from Applied Energy)
These findings are described in the article entitled Standardized Matrix Modeling of Multiple Energy Systems, and Optimal configuration planning of multi-energy systems considering distributed renewable energy recently published in the journal IEEE Transactions on Smart Grid, the article entitled Mixed-Integer Linear Programming-Based Optimal Configuration Planning for Energy Hub: Starting from Scratch, and Automatic and linearized modeling of energy hub and its flexibility analysis, recently published in the journal Applied Energy.
This work was conducted by Yi Wang (Tsinghua University), Ning Zhang (Tsinghua University), Wujing Huang (Tsinghua University), and Chongqing Kang (Tsinghua University), Daniel Kirschen (University of Washington).