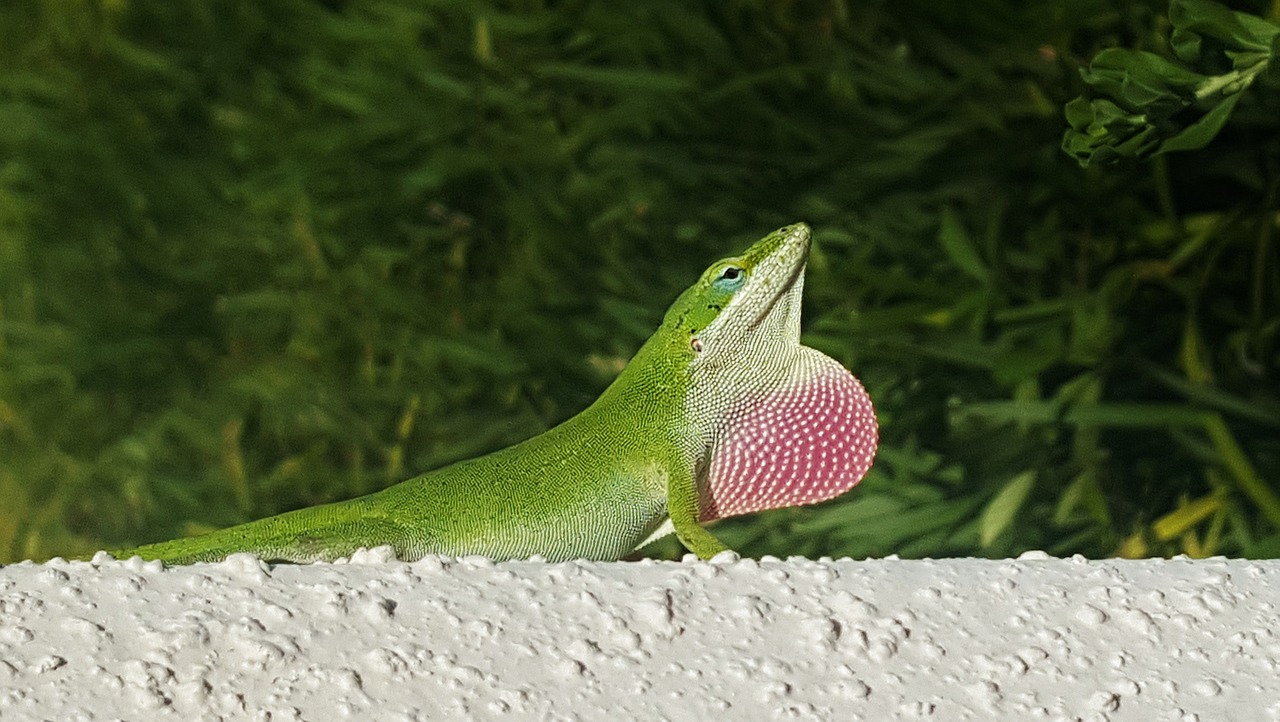
Despite recent advances in regenerative medicine, re-growing a lost limb is an insurmountable feat for human beings, confined for now to science fiction and comic book superheroes. For mammals, loss of appendages results in the formation of scar tissue with little to no regeneration, leaving victims of traumatic accidents or birth defects with limited options for treatment and care.
In order to approach this problem, scientists have turned to studying model organisms that undergo robust regeneration, like hydra, planarian worms, zebrafish, and axolotl salamanders. However, while we have learned valuable lessons about regeneration from these animals, they all have one thing in common: they are anamniotes — animals that can only lay eggs in water — and are more distantly related to us than amniotic organisms, an evolutionary group that includes reptiles, birds, and other mammals.
Lizards, which are reptiles, are evolutionarily the closest vertebrates to humans that can regenerate an entire appendage, and thus make a great model for research. Many lizard species have the ability to autotomize (self-amputate) their tails in response to predation and regenerate a whole new replacement. This process has been studied in great detail in Anolis carolinensis, the green anole, native to the southeastern United States.
Tail regeneration is not an immediate process; it takes months for a tail to fully regenerate, and it involves a highly orchestrated, dynamic series of events. Immediately after autotomy, a wound epithelium covers the injury site and protects it from infection. Over the next 30-60 days, the regenerated tail elongates, and distinct tissues become apparent. Whole muscle groups start forming, and a cartilage tube replaces the lost vertebrae. Newly-formed vasculature provides the regenerated tail with oxygen and nutrients, while nerves descend and make connections with the muscle. Finally, the tissues mature, giving rise to a functional tail.
Although we understand the sequence of events that take place during anole tail regeneration, many questions remain unanswered: why can lizards regenerate whole new muscle groups and cartilage while we mammals cannot? What is the source of cells that make new tissues in a regenerating tail? What genes are driving this process? Importantly, what can lizards tell us that we can apply to human regenerative medicine?
A team of scientists at Arizona State University sought to answer those questions by looking at adult muscle stem cells, called satellite cells. These cells, which are present in virtually all vertebrates, are found dormant in our skeletal muscles. After an injury to the muscle, as might result from an accident or an intense workout, these cells sense the damage and become activated. They rush to the site of injury and help repair it by fusing with each other to make new muscle fibers. However, repairability is limited in mammals, and satellite cells cannot cope with the extensive muscle damage caused by chronic diseases like muscular dystrophies or traumatic accidents. This is in stark contrast to what we see in lizards, where after losing a tail, they regenerate completely new, fully functional muscle groups.
In trying to understand how lizard satellite cells might be different, a transcriptomics approach was used to compare mouse, human, and lizard satellite cells. Transcriptomic analyses enable scientists to look at all of the genes that are active in a particular tissue or cell and compare them to other tissues and cells in order to understand the biological processes at play. Normally, comparing across different species is not feasible, but the use of a new algorithm, known as XGSA (Cross-species Gene Set Analysis) allowed for a comparison of gene expression levels.
The findings were surprising: despite the difference in regeneration ability, lizard satellite cells were very similar to mouse — and even human — satellite cells, in terms of global gene expression. However, after digging deeper and examining individual genes, key differences emerged. While both cell types expressed genes associated with muscle development, maintenance, and repair, lizard satellite cells also expressed a unique set of genes involved in cartilage formation. This suggested that lizard satellite cells might participate not only in muscle regeneration but possibly in cartilage formation as well — which mammalian satellite cells cannot do.
To test this theory, satellite cells from mice and lizards were grown in dishes, in 3-D micromasses. After a week, the cells were inspected using microscopy, gene expression analysis, and immunocytochemistry techniques. As expected, the mouse satellite cells expressed proteins necessary for muscle formation and fused with each other into muscle fibers. The lizard satellite cells fused to make some muscle fibers, but many of the cells aggregated in dense cartilage nodules. When gene and protein expression was examined, lizard satellite cells were found to express high levels of cartilage-specific genes and proteins, unlike mouse satellite cells.
These findings agreed with the transcriptomic data and suggested that while mouse satellite cells are monopotent and can only become muscle, lizard satellite cells can participate in both muscle and cartilage repair. This key difference might help explain, in part, the discrepancy in regeneration ability between the two species. Hopefully, further research into the topic will shed light on new strategies for approaching the problem of human tissue engineering and regeneration.
Regenerating complex structures requires a variety of different tissue types, like cartilage, muscle, nerves, and skin to form at the right time, and in the right location. Additionally, each individual tissue must properly communicate and integrate with the others, in order to function as a whole. For the time being, re-growing a lost limb remains a daunting task, but the hope is that lessons from lizard satellite cells will provide important pieces of the puzzle.
These findings are described in the article entitled Identification of satellite cells from anole lizard skeletal muscle and demonstration of expanded musculoskeletal potential, recently published in the journal Developmental Biology. The research was conducted by Joanna Palade, Elizabeth D. Hutchins, Rajani M. George, John A. Cornelius, Alan Rawls, Kenro Kusumi, and Jeanne Wilson-Rawls from Arizona State University, and Djordje Djordjevic and Joshua Ho from the Victor Chang Cardiac Research Institute.