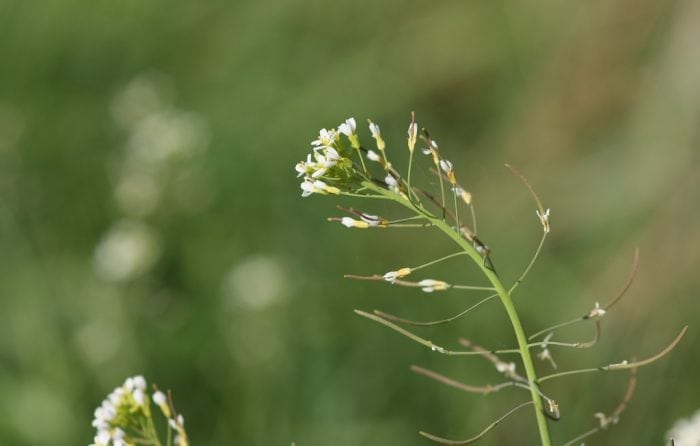
The term auxin is derived from the Greek word “auxein,” which means to grow or to expand and was sealed by Charles Darwin more than a century ago. In “The Power of Movement in Plants” (1880), Darwin first described the effects of light on the movement of canary grass coleoptiles. He demonstrated that the tip of the seedling is responsible for producing a movable signal, auxin, which is transported to the lower part of the coleoptile where, following the light stimulus, bending occurs. Auxin is probably the most intensely-studied molecule in plants as it impacts virtually every aspect of growth and development during their life cycle.
The role of auxin is warranted by the coordination of its synthesis, metabolism, transport, and perception. The plant cell traduces the auxin signal through a well-characterized nuclear signaling pathway, triggering transcriptional responses depending on a specific cell, tissue, or organ.
Auxin signaling pathway initiates once the hormone moves into the nucleus and is bound by a coreceptor system build by one of the E3 ubiquitin ligases SCFTIR1/AFBs and their degradation substrates AUX/IAAs transcriptional repressors. Upon auxin binding SCFTIR1/AFBs trigger ubiquitylation and further AUX/IAA turnover by the proteasome. Given AUX/IAAs block the expression of auxin-responsive genes, their degradation is essential for auxin pathway activation.
Since plants are sessile organisms unable to escape changes in the environment, the degradation of pre-synthesized AUX/IAA repressor proteins instead of the “de novo” synthesis of activation proteins constitutes a more rapid and efficient strategy for the activation of molecular pathways required to adapt to new situations. Thus, the ubiquitin-proteasome system via the exquisite action of specific E3 ubiquitin ligases, such as the SCFTIR1/AFBs, recruit directly proteins for targeted degradation. SCF-type E3 ligases are the most abundant substrate recognition complexes in eukaryotic cells and have been implicated in every major phytohormone signaling pathway. Each individual SCF E3 ligase is a multimer consisting of a scaffold protein Cullin 1, a RING RBX1 for binding an E2 conjugating enzyme loaded with ubiquitin, and a substrate binding module build by the adaptor protein SKP1 (in Arabidopsis ASK1) and, an interchangeable substrate-recognition unit F-box Protein (FBP).
In the last decade we have gained tremendous knowledge of how the signal auxin is perceived and transmitted, and now we are starting to unveil a new level of regulation of the system at the level of SCFTIR1/AFBstability. Since the Arabidopsis genome encodes hundreds of FBPs, and ASK is able to associate with diverse FBPs to form multiple SCF complexes, the challenge of regulating SCF assembly is particularly relevant. The SCF complex is, therefore, an exceptional core in which different levels of post-translational modifications might take place. In addition to auxin, nitric oxide (NO) is considered a ubiquitous signal in plants which contributes to determining the morphology and developmental pattern of roots, in part by the modulation of auxin response. Previously, we gained evidence on the role of the second messenger NO for the regulation of the FBP TIR1 for auxin sensing. We wondered further whether NO might play a broader role in regulating the SCFTIR1/AFB and its functionality in plant cells.
One mechanism by which gaseous NO modifies the fate of proteins is through redox modification of cysteine residues (S-nitrosylation). Interesting is the fact, that S-nitrosylation can lead to changes in protein localization, protein activity or protein-protein/ DNA-protein interaction. This constitutes a potent redox signal in many physiological processes in animals, humans, and plants, where a rapid and versatile regulation is needed.
In our recent studies, we have demonstrated NO directly regulates SCFTIR1E3 ubiquitin ligase assembly through S-nitrosylation of ASK1. NO triggers the redox modification of 2 cysteines in ASK1 improving the interaction between TIR1 and the SCF scaffold CUL1-RBX, consequently impacting auxin signaling activation. Collectively, our results substantiate the interplay between two post-translational modifications, S-nitrosylation, and ubiquitylation as mean for robust fine-tuning of the auxin receptor machinery, which dictates physiological responses during plant growth and development.
Since SCF E3 ubiquitin ligases play a fundamental role in the sensing and signaling triggered by various phytohormones, NO can probably impinge on those as a versatile second messenger. Ours is the first evidence of NO directly regulating SCFs in plants, and probably this constitutes just the tip of the iceberg for NO involvement in the ubiquitin machinery. Interestingly, in animals, S-nitrosylation has been shown to be important for the regulation of the ubiquitin-proteasome system activity associated with neurodegenerative diseases. The universality of both S-nitrosylation and ubiquitylation associated with proteostasis provides a valuable platform to extend this study to other eukaryotic organisms.
Findings on the role of NO on SCF-E3 ligases was recently published in the Redox Biology journal in the article entitled Regulation of SCFTIR1/AFBsE3 ligase assembly by S-nitrosylation of Arabidopsis SKP1-like1 impacts on auxin signaling.
This work was conducted by María José Iglesias, María Cecilia Terrile, Natalia Correa-Aragunde, Silvana Lorena Colman, Diego Fernando Fiol, Ramiro París, Lorenzo Lamattina, and Claudia Anahí Casalongué from the Universidad Nacional de Mar del Plata (Argentina), Alicia Izquierdo-Álvarez from the Hospital Universitario de La Princesa (Spain), Nuria Sánchez-López and Anabel Marina from the Centro de Biología Molecular “Severo Ochoa” (Spain), Luz Irina A. Calderón Villalobos from Leibniz Institute of Plant Biochemistry (Germany), Mark Estelle from the University of California San Diego (USA), and Antonio Martínez-Ruiz from the Centro de Investigación Biomédica en Red de Enfermedades Cardiovasculares (Spain).