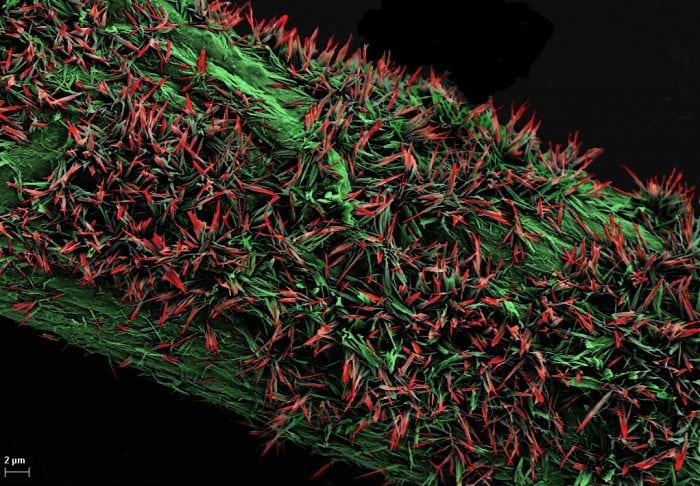
Chitosan, the second-most abundant natural polysaccharide, is a cationic polymer widely used for the preparation of particles, hydrogels, or scaffolds intended for biomedical purposes [1,2].
All these intensively studied platforms are typically formed by electrostatic interaction between a positively charged chitosan and negatively charged complexing agent. Their biocompatibility, biodegradability, as well as tunable properties, make chitosan-based complexes a versatile tool utilizable in distinct nanomedical applications [3].
The attractiveness of these systems is, moreover, underlined by the fact that their fabrication is generally seen as simple, convenient, and controllable [3,4]. Given the huge potential of chitosan-based complexes for clinical translation, it is not surprising that they have experienced an upsurge in interest, as evidenced by an explosive growth in the number of in vitro and in vivo studies addressing specific medical problems.
The principal problem is, however, that chitosan is not cationic (nor soluble) throughout the whole pH range. It has been known for more than two decades that the pKa of chitosan is around 6.3-6.5, depending chiefly on its molecular weight and degree of deacetylation [5]. Since chitosan behaves as a proton acceptor at pH below the pKa, chitosan is highly protonated, resulting in a sufficient amount of positive charge facilitating electrostatic interaction with negatively-charged complexing agents, generating stable polyelectrolyte-based complexes. In this respect, chitosan-based complexes are typically prepared under acidic conditions. On the other hand, at pH above pKa, there is massive deprotonation, making chitosan almost bare of cationic charge and unable for electrostatic interactions. Given the pH-dependent degree of chitosan protonation, the physico-chemical properties of resultant polyelectrolyte complexes are obviously variable.
Although chitosan-based complexes have been tested in a huge number of in vitro and in vivo studies to date [3], it is striking that none of these studies has been interested in the pH-dependent characteristics of these complexes, especially after exposure to physiological pH of around 7.4, i.e. pH > pKa. In this context, Mazancova et al. 2018 [6], asked fundamental and speculative questions relevant to the understanding of the behavior of chitosan-based complexes: i) Do chitosan-based polyelectrolyte complexes exist at physiological pH? ii) What is the composition of chitosan-based complexes transferred to an environment with pH different from that at which they were prepared?
On the example of model chitosan/tripolyphosphate (TPP) complexes, Mazancova et al. provide a solid evidence that upon exposure to physiological pH or pH generally higher than that used during complex fabrication, chitosan/TPP complexes undergo a gradual dissociation into free chitosan molecules, that are, moreover, phase-separated. The transition is accompanied by the release of a corresponding amount of TPP as a direct consequence of the decrease in chitosan protonation at elevated pH. Simply, chitosan/TPP complexes, typically prepared at acidic pH, do not exist under physiological conditions, as they spontaneously dissociated into phase-separated chitosan chains with only a residual amount of bound TPP.
This finding is seen as a paradigm shift in understanding of chitosan-based systems not only in the context of correlation with biological data but, more importantly, with regard to their overall translational potential. It is worth noting that any application of chitosan-based complexes under in vitro and in vivo conditions should be carried out with the warning that their original properties will be completely altered.
These findings are described in the article entitled Dissociation of chitosan/tripolyphosphate complexes into separate components upon pH elevation, recently published in the journal Carbohydrate Polymers. This work was conducted by Petra Mazancová, Veronika Némethová, Dušana Treľová, Lucia Kleščíková, Igor Lacík, and Filip Rázga from the Polymer Institute of the Slovak Academy of Sciences.
References:
- Jayakumar, R., et al., Biomedical applications of chitin and chitosan based nanomaterials—A short review. Carbohydrate Polymers, 2010. 82(2): p. 227-232.
- Jayakumar, R., M. Prabaharan, and R.A. Muzzarelli, Chitosan for biomaterials I. 2011: Springer.
- Bugnicourt, L. and C. Ladavière, Interests of chitosan nanoparticles ionically cross-linked with tripolyphosphate for biomedical applications. Progress in Polymer Science, 2016. 60: p. 1-17.
- Agnihotri, S.A., N.N. Mallikarjuna, and T.M. Aminabhavi, Recent advances on chitosan-based micro- and nanoparticles in drug delivery. Journal of Controlled Release, 2004. 100(1): p. 5-28.
- Roberts G.A.F. Chemical Behaviour of Chitin and Chitosan. In: Chitin Chemistry. 1992: Palgrave.
- Mazancová, P., et al., Dissociation of chitosan/tripolyphosphate complexes into separate components upon pH elevation. Carbohydrate polymers, 2018. 192: p. 104-110.