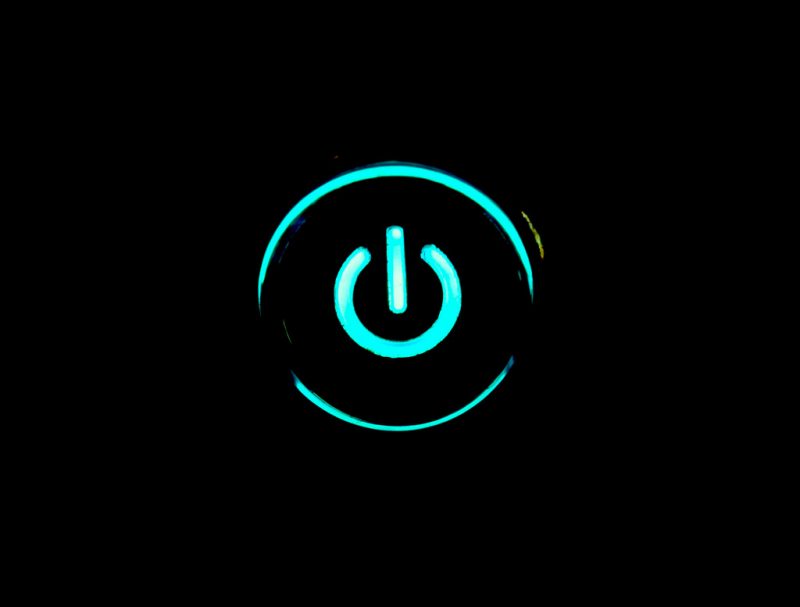
Glycosylation is the ubiquitous, highly-regulated process by which carbohydrate is added to proteins and lipids to form glycoconjugates (glycans). These carbohydrate portions of glycans can be as simple as a single sugar but are frequently more complex, forming linear and branched polysaccharide structures from a limited number of sugar units, such as galactose, N-acetylglucosamine, mannose, fucose, and sialic acids.
Glycans are synthesized in a stepwise fashion, with an activated sugar (a sugar covalently attached to a nucleotide) donating its monosaccharide unit to the glycan acceptor, in reactions that are catalyzed by enzymes known as glycosyltransferases that are typically located in the subcellular compartments of the Golgi. Nascent protein enters the Golgi from the endoplasmic reticulum, where it encounters a variety of enzymes in each Golgi stack, where it is progressively modified before being exported elsewhere in the cell, or released extracellularly. The biological functions of glycans are wide-ranging, encompassing structural, immunological, and regulatory roles, including modulation of enzyme activities. Some examples of glycans are shown in Figure 1.
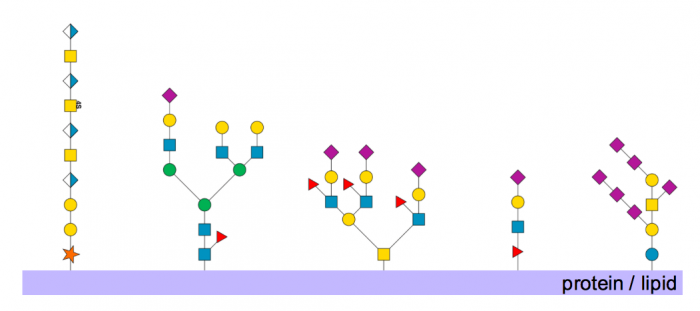
Figure 1. Examples of glycans synthesized in the Golgi. From left to right: dermatan sulfate; N-linked glycan; O-linked glycan; O-fucose glycan; a ganglioside. Published with permission from Andrew Mc Donald.
A key concept in this process, which applies to all of metabolism, is that of a steady state. In a steady state, the rates of formation of a molecule, whether it be through transport into a compartment or through synthesis, are balanced by the rates of its removal, either through further catalysis or transport out of that compartment. If the system is perturbed, by the entry of some external metabolite, say, but then returns to the same steady-state level, that steady state is said to be stable. If, after such a disturbance, the system does not return to the old level but finds a new steady state, then the original steady state is unstable.
A second concept fundamental to metabolism is that of the saturable nature of enzymes. In general, most enzymes obey Michalis-Menten kinetics, in which the initial rate of formation of product depends in a hyperbolic fashion on the initial substrate concentration: increasing the concentration of the substrate decreases the amount of free enzyme available to bind, and the initial rate tends towards an asymptote, or maximal velocity (Vmax). The concentration of substrate at which the rate of catalysis is at half of the maximum is known as the Michaelis constant, or Km.
A competitive inhibitor of an enzyme binds at its active site in such a way that it prevents substrate binding while it is bound. This increases the effective Km value of the enzyme, so that, in the presence of inhibitor, a higher concentration of substrate is needed in order to saturate the enzyme. This is one way by which pharmaceuticals can be designed to inhibit enzymes involved in the mechanism of disease, by mimicking their natural substrates and temporarily lowering their activities. The effect might be two-fold in nature: decreasing the activity of the enzyme will disturb the steady-state, potentially causing both an increase in the substrate concentration and decreasing the concentrations of products downstream. In glycosylation, the enzymes are specific for a particular pattern of sugars in the glycan substrate and hence there may be many distinct substrates that compete with one another for the active site, which increases the apparent Km.
With some enzymes, the initial rate reaches a peak, as substrate concentration is increased, but then is observed to fall off at higher substrate concentrations, a phenomenon called high-substrate inhibition. Several glycosyltransferases show this behavior, where they become inhibited by their own substrates at higher concentrations.
In a recent theoretical study, we proposed a mechanism by which the glycan acceptor binds to the enzyme first, but another glycan substrate (or else the same glycan) binds reversibly at another site to form a non-productive enzyme-substrate-substrate (ternary) complex. In a thermodynamically open system, this has an interesting consequence that for certain values of an external parameter, such as the diffusion constant (which controls rate of entry and exit of substrates from the system) there can exist up to three steady states for the concentration of the substrate: “low,” “middle,” and “high,” with the middle state being unstable and the low and high states being stable.
A plot of the steady states of the substrate concentration against the value of an external parameter shows an S-shaped curve, as illustrated in Figure 2. Since two steady states are concurrently stable over a range of parameter values, the system exhibits the phenomenon of bistability. A feature of bistable systems is that they can act as on/off switches: after a stimulus, a sudden jump can occur from one stable branch to the other. Looking at the figure, moving rightward along the lower stable branch, one reaches a limit point (LP), where the stable and unstable branches meet; the system jumps to a new stable steady state, on the upper branch, as shown by the dashed line. A similar event occurs when moving leftward along the upper branch, which switches the system to the “off” state, where the substrate is maintained at a low level.
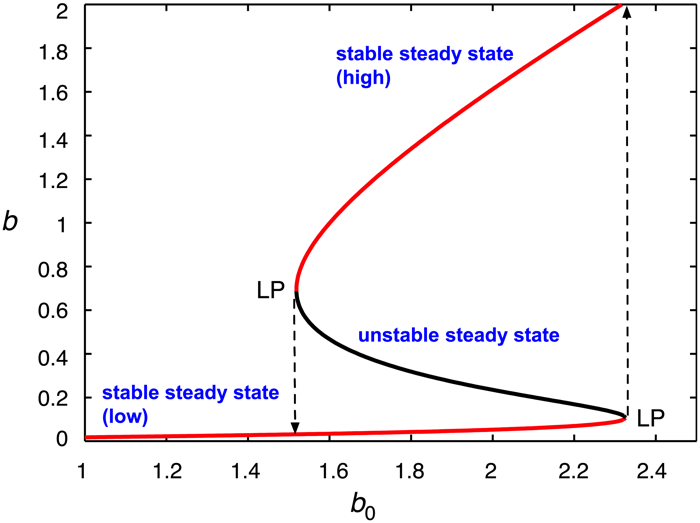
Figure 2. Switching between concurrently stable steady states as a result of enzyme inhibition by high concentrations of substrate. [Original source: A mechanism for bistability in glycosylation (McDonald et al., 2018).] Republished with permission from PLOS.
The ability to switch glycosylation pathways would have implications for the types of glycan that are expressed on proteins. Glycans express epitopes that are involved in cell-cell recognition and signaling, which, if altered, could result in physiological changes. Cancer malignancy and tumor progression, as well as bacterial defense and immune responses, are known to coincide with altered glycosylation. There are implications for biotechnology, too, in the production of therapeutic antibodies in non-human cell lines, to tailor their carbohydrate components to display human-like characteristics.
Common to all of these applications is the possibility of hidden states that may not be realized until the system has been perturbed by an internal or external noise source. What this result also highlights is the need for further research into the kinetics of the glycosyltransferases, in order to obtain estimates of the kinetic parameters, including those contributing to substrate inhibition, so that better predictions can be made of healthy and diseased states.
These findings are described in the article entitled A mechanism for bistability in glycosylation, recently published in the journal PLoS Computational Biology. This work was conducted by Andrew G. McDonald, Keith F. Tipton, and Gavin P. Davey from Trinity College Dublin.