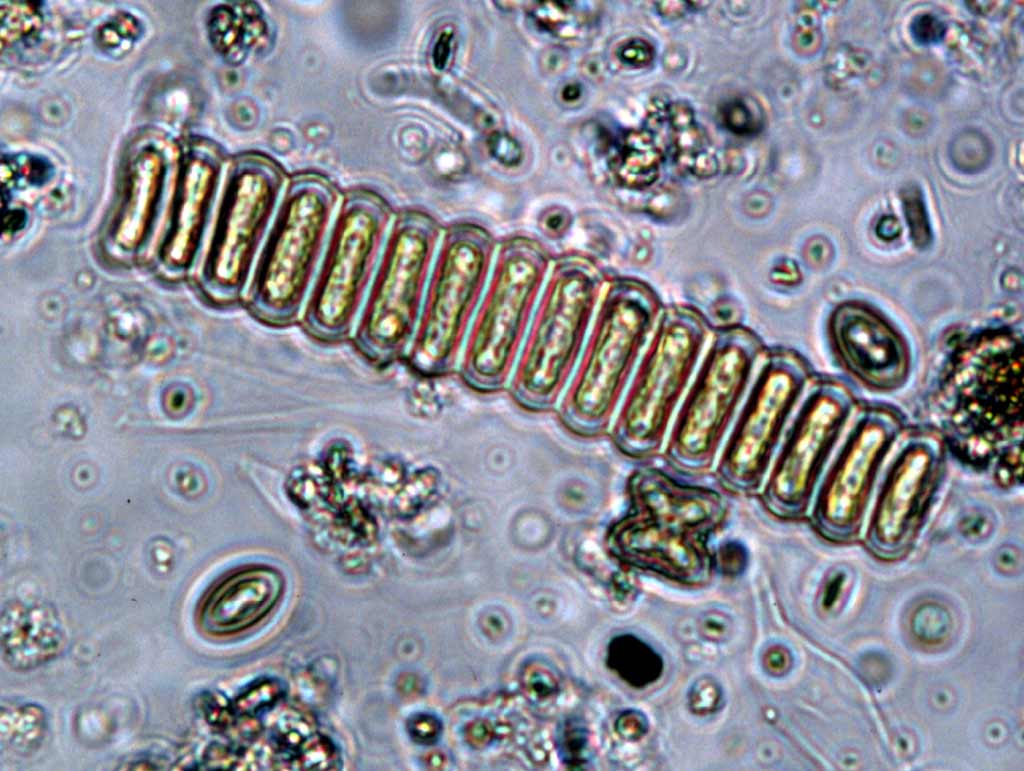
The energy crisis, water shortage, and pollution are among the major challenges confronting sustainable environmental existence. Renewable energy derived from sustainable feedstocks can reduce the load on fossil fuels and curb greenhouse gas (GHG) emissions. Biofuels, particularly bioethanol and biodiesel, have been touted as one of the most propitious and greener alternatives since the beginning of the millennium. In this regard, algal-derived biofuels have the potential to become the savior of our dwindling fossil fuel reserves.
Algae are a diverse group (~100,000 strains) of photosynthetic organisms ranging from cyanobacteria (microalgae) to giant kelp (macroalgae). Microalgae harness CO2 and sunlight from the atmosphere to synthesize biomolecules including proteins, carbohydrates, lipids, and value-added products (carotenoids, vitamins, and sterols). The energy-rich compounds, particularly intracellular lipid reserves, serve as potential raw material for biodiesel production; the carbohydrates accumulated can be converted to bioethanol by simple fermentation, and the algal value-added products can be alternate sources of nutraceuticals. Furthermore, microalgae can capture CO2 and mitigate heavy metals from the environment and industrial waste streams, providing an eco-friendly impact.
Despite microalgae having great potential for biofuels and mitigation of heavy metals, its utilization on a large scale is still a long road ahead. The key factors that could expedite the economical production of biofuel production and metal bioremediation include increasing the algal biomass productivity, utilization of copious/abundant feedstocks, and cost-effective extraction of lipids/carbohydrates, along with the utilization of residue biomass for high-value products. Utilization of seawater or brackish water that is not suitable for irrigation resolves the algal farming dilemma as there is no competition with the fresh water reserves, land, and nutrients to cultivate these microorganisms.
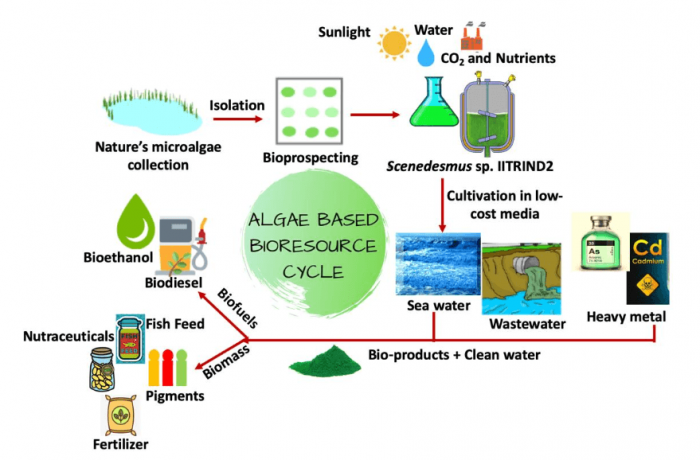
Figure courtesy Neha Arora.
To this end, our research efforts were focused toward bioprospecting of high lipid accumulating microalgal strains from a local lake capable of growing in seawater. Among the isolated strains, Scenedesmus sp. IITRIND2 was able to tolerate natural seawater (35 g/L sea salts), showing rapid growth rate along with an accumulation of high lipid and carbohydrate content. A series of physiological and biochemical studies at both lab and pilot scale (in photobioreactors operated under summer outdoor conditions to mimic the real temperature and light fluctuations) provided detailed insights into the seawater acclimatization characteristics of the microalga. Such an adaptation was attributed to the increase in neutral sugars associated with structural and storage functions including glucose, mannose, galactose, fucose, and ribose.
The carbohydrate rearrangements may aid in rewiring the cellular components and membrane permeability in circumventing the detrimental effects of high salinity. Further, the applicability of biodiesel in conventional diesel engines was verified by analyzing its fuel properties using the American and European Union fuel standard protocols.1,2
We then set out to understand something that is a key interest to the algal biologists and industries: how precisely a fresh water microalga adapted to such high saline conditions. We designed a systematic workflow to decipher the changes in the microalga occurring at the gene, protein, and metabolite levels when cultivated in sea water as opposed to fresh water. The results showed that the microalga remodeled its membrane permeability by restricting ion channels, decreasing the surface potential and excreting extra polysaccharides as compared to fresh water medium.
Interestingly, an increase in cell size along with disorganization of cellular structure with large lipid accumulation and few starch granules in 100% ASW culture were visualized in electron micrographs of the microalga as compared to the control cells. Based on an “integrated omics approach” comprised of proteomics, metabolomics, and lipidomics, a salinity-driven metabolic pathway was hypothesized which aided in development of a metabolic model and in identifying genetic targets for engineering non-halotolerant algal species.3
The next challenge was to utilize this particular microalga for remediation of toxic heavy metals present in the environment. This led us to the development of a synergistic modus operandi for mitigating heavy metals such as arsenic and cadmium along with biodiesel production. Scenedesmus sp. IITRIND2 showed an exceptional capacity to tolerate 500 mg/L of both the arsenic forms (III, V) and 100 mg/L of cadmium. A high removal efficiency of 87 % for arsenic (III, V) and 95% for cadmium was recorded at an initial metal concentration of 50 mg/L in a cultivation medium mimicking well water. The microalga also showed ~ 40-45% lipid content of dry cell weight when cultivated under the heavy metal stress. The biodiesel produced from the lipids also complied with the international biodiesel standards, thereby indicating the feasibility of such a hybrid approach.4,5
Indeed, we hope to eventually to go one step further by exploring the potential of Scenedesmus sp. IITRIND2 in open raceway ponds on Indian soil using real wastewaters and leachates. A small step toward this is being initiated by our group by cultivating this microalga on local diary wastewater obtained from Indian Institute of Technology Roorkee campus. The results obtained in terms of bioremediation of the wastewater are very promising with a removal of ~ 75% total organic carbon, ~ 94% chemical oxygen demand, ~ 90% total nitrogen and ~ 93% total phosphorous along with mitigation of chlorine (~ 91%), fluorine (~ 89%) and bromide (~ 66%), respectively, in just 10 days of cultivation. The microalga also accumulated high intracellular lipids and carbohydrates which were converted into biodiesel and bioethanol.
In summary, we systematically addressed a few of the commercialization barriers to algal farming, including testing sea water as cultivation medium, developing a metabolic model, and integrating biofuel production with wastewater and heavy metal remediation. These concerted efforts are focused towards the initialization of large-scale microalgae cultivation for biofuel and bioremediation which can promote its sustainable application in the near future. Indeed, robust and highly productive microalgal strains such as Scenedesmus sp. IITRIND2 could be an answer to save the planet from the energy crisis, climatic deterioration, and potable water contamination. We believe our study could be a foundation step towards the realization of an algal-based bio-economy and subsequently help algae become the “green mines” fuelling the world’s needs.
These findings are described in the article entitled Elucidating the unique physiological responses of halotolerant Scenedesmus sp. cultivated in sea water for biofuel production, recently published in the journal Algal Research, as well as the other works listed below.
References:
- Arora, N. et al. Insights into the enhanced lipid production characteristics of a fresh water microalga under high salinity conditions. Ind. Eng. Chem. Res. 56, 7413–7421 (2017).
- Arora, N. et al. Elucidating the unique physiological responses of halotolerant Scenedesmus sp. cultivated in sea water for biofuel production. Algal Res. 37, 260–268 (2019).
- Arora, N. et al. Delineating the molecular responses of a halotolerant microalga using integrated omics approach to identify genetic engineering targets for enhanced TAG production. Biotechnol. Biofuels 1–17 (2019). doi:10.1186/s13068-018-1343-1
- Arora, N. et al. NMR-based metabolomic approach to elucidate the differential cellular responses during mitigation of arsenic (III, V) in a green microalga. ACS Omega 3, 11847–11856 (2018).
- Arora, N. et al. A hybrid approach integrating arsenic detoxification with biodiesel production using oleaginous microalgae. Algal Res. 24, 29–39 (2017).