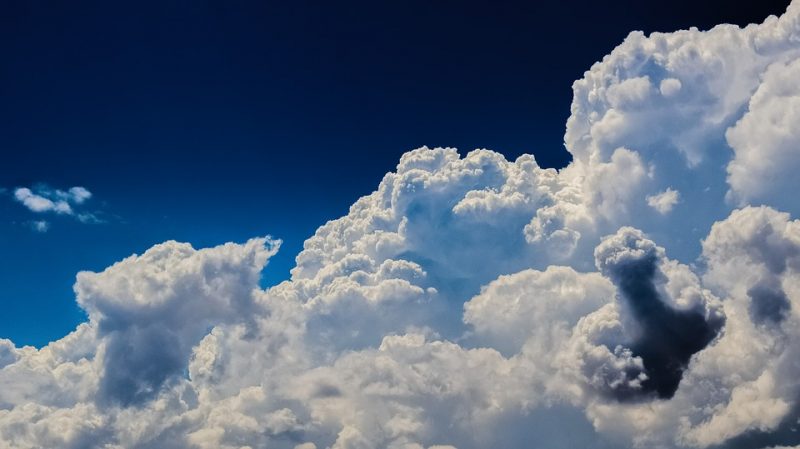
Above about four kilometers up in the atmosphere, clouds begin to contain both liquid droplets and ice crystals. Because two phases of water coexist, a variety of interesting small-scale processes can occur in the cloud: droplets can coalesce and ice crystals can aggregate or droplets can collide with ice crystals and freeze or ice crystals can melt as they sediment out of the cloud. These processes are jointly called cloud microphysics and can be quite difficult to model.
Ice crystals are especially challenging because they have a range of geometries from dendritic to plate or needle. At higher altitudes, they form thermodynamically by nucleation when the air is supersaturated with water vapor. Generally, there is also a particulate surface from dust or soot or bacteria that lowers the free energy barrier for phase change. We have been interested in modeling a set of other mechanical ice formation pathways that occur at warmer temperatures. Within the complicated world of cloud microphysics, these pathways are referred to as secondary ice production.
Secondary ice production relies on collisions to cause shattering. For example, if a droplet and an ice crystal collide, the droplet may start to freeze, releasing latent heat and causing a pressure build-up that eventually shatters the freezing droplet. Or if two large ice crystals or graupel particles collide, their impact may be enough to splinter the two into many tinier pieces, something akin to Bill Watterson’s PIFF! above that splatters the snowball everywhere.
To approximate how many ice crystals could be formed from these processes, we built a model that tracks a parcel of air as it rises through the atmosphere. Our simulations include droplet formation and ice nucleation along with secondary ice production. With the model, we find that, in a kind of Goldilocks zone where the upward speed of the parcel and its initial temperature are just right, secondary ice can generate 1000 times as many crystals as nucleation alone.
The representation of ice crystal geometries is also crucial. If we assume that ice crystals are spheres, that 1000-fold enhancement drops dramatically. But if we model the ice crystals more realistically, with elongated geometries like the variety of dendrites and needles actually seen in the atmosphere, the ice crystal number enhancement comes back.
Such a large, additional source of atmospheric ice crystals will have hydrological and radiative impacts on climate. Often precipitation over landforms from the ice phase: ice crystals grow at cold temperatures and then fall out of the cloud to melt and form rain. So representing secondary ice production in models could yield better simulations of precipitation under certain conditions. Or more and smaller ice crystals in a cloud may yield a warming effect because the crystals are mostly transparent to incoming solar radiation and mostly opaque to outgoing terrestrial radiation.
These kind of large-scale impacts are the impressive part of cloud microphysics: all the small PIFFs! of droplets and ice crystals four kilometers up can actually alter the temperature and rain we see at the Earth’s surface.
This study, Investigating the contribution of secondary ice production to in-cloud ice crystal numbers was recently published in the Journal of Geophysical Research: Atmospheres.