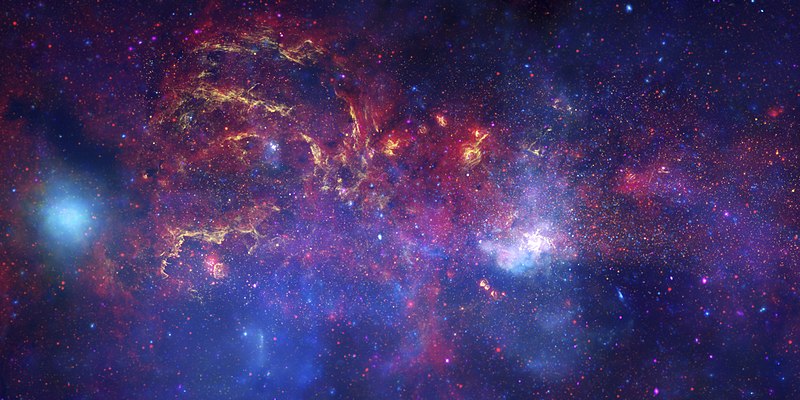
A typical galaxy contains billions and trillions of stars, like our closest neighbor, the Sun. In the field of Cosmology, a single galaxy is considered to be the unit quantity to study the vast and amazing Universe. With bigger and better telescopes, we are able to observe and catalog millions of such galaxies and quasars (very bright “active” galactic centers). If we were to observe the Universe around us, at large distances, more or less the distribution of these galaxies appears to be isotropic — which means that there is no preferred direction in the Universe. Another way we know this isotropy exists is because we are surrounded by uniformly-distributed light from the early Universe in all directions (precisely speaking, microwave radiation known as the Cosmic Microwave Background (CMB)).
Also, there is no reason to believe that we are in any special place in the Universe, as our galaxy is no more than a speck of dust in the vast multitude of galaxies just like any other one. If we assume that we are not in any special location in the Universe to observe the isotropy, meaning that there is no preferred location in the Universe, then all the locations are treated on equal footing. This is also called the Copernican principle. Observationally, it is difficult to “prove” this assumption. We observe on the past lightcone at a single cosmological time, which means that we can only directly test isotropy based on our worldline (that which traces our path in the spacetime). Since isotropy about all galaxy worldlines implies homogeneity, and we cannot observe all the worldlines, we adopt a Copernican Principle (i.e. our worldline is not special) and we deduce the so-called “Cosmological Principle” on the basis of isotropy we observe. When we combine this principle with a theory of gravity like general relativity and bring in the ingredients that we know based on fundamental physics, we get the standard model of the Universe.
The most widely-accepted model of the Universe is called the Lambda CDM model. This model is based on the existence of two exotic ingredients that we have not directly observed in a laboratory, namely dark matter & dark energy. According to this model, only 4% of the observed Universe is made of the matter and energy we know of, and the rest of the 96% is unknown. Dark matter is an elusive, (almost) all-pervading, a non-baryonic substance that interacts only through gravity. Direct detection and laboratory study of dark matter is, therefore, an open challenge as is to find a suitable dark matter candidate from particle physics. This situation is reminiscent of the quest for the aether medium in the past century. Dark energy, on the other hand, could be motivated from a cosmological constant (the purported biggest blunder of Einstein that came to the rescue). Unfortunately, the cosmological constant is so small in comparison (of the order of ~10−120) to the expectation from another successful field of physics known as the Quantum Field Theory. This discrepancy is even called the worst theoretical prediction in the history of physics!.
As we know, the development of science is based on direct observations of the hypothesis formed. As Richard Feynman nicely put it — no matter how beautiful a theory is, if it does not match with the experiments, it is wrong. Apart from this uncomfortable situation of not knowing the major part of the Universe, there are also few observational inconsistencies that irk cosmologists in whole-heartedly supporting the standard model of the Universe. We also know that general relativity is not reconciled with quantum mechanics (another observationally strong field of physics). So, in principle we could be looking at alternative models of gravity as well, to look beyond general relativity (which is leading us to the standard model). Hence, there is a growing interest in extending cosmology beyond the standard Lambda CDM model.
It is, therefore, essential that any observational study of the Universe (like any other branch of science) is independent of the biases we may have from learning different theoretical models. So, development of model-independent observational techniques can lead us to a better understanding of the Universe by pointing towards exploring superior theoretical models. At least, the observational analysis should be open to testing a multitude of potential theoretical models.
For example, we observationally notice all the galaxies are moving away from us based on the shift in the frequency of light known as redshift. This is why we believe that the Universe is expanding. To calculate the distance of these galaxies are from us, we need to assume some theoretical model of the Universe which dictates its evolution a priori. For e.g., we think the Universe is expanding at a rapid rate in recent times (accelerating) only because we assume the standard model scenario. The Universe could as well have been expanding linearly since its inception and the observational data of the so-called standard candles (supernovae) can still be consistent. Theoretically speaking, the Universe could be of different geometries containing ingredients in various proportions having a bunch of possible evolution scenarios along with different alternative theories of gravity. Therefore, any code/calculation that analyses observations should have the possibility of analyzing the data through many different theoretical models. The code “correlcalc” is an effort in this direction. It is made to analyze the galaxy catalog data containing redshift and directional positions of all the observed galaxies, in light of various possible theoretical models.
At the beginning of this article, we discussed how the Universe roughly appears the same in all directions at large distances. At smaller scales, (of the order of up to 100Mpc) gravity pulls matter closer together forming some structures. All the structures in the Universe we observe today are put together by the gravitational pull. So, by observing how different the Universe appears at large scales in comparison to a totally random distribution, we can extract some key information and statistical parameters. These help in putting constraints on the theories of gravity and evolution of structures etc.
In the figure below, notice how the filaments, voids and other structures can be seen in the observed patch of sky (at a particular redshift slice – shown on the right).
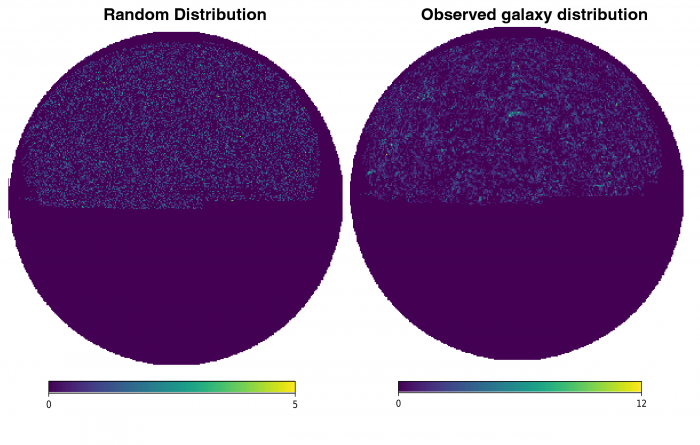
Image courtesy Rohin Kumar
If we are assuming an alternative model for the evolution of the Universe (with or without different gravity models) and analyze the data, if extracted parameters are self-consistent with the alternative model, it makes a much more compelling case as the standard model (at least from this set of observations). In a sample study conducted based on the correlcalc code using data taken from Sloan Digital Sky Survey (SDSS), we concluded that observations are as consistent with a linearly coasting model of the Universe in the same way as they are for the standard model. Hence we strongly believe more efforts are needed to (in)validate the potential alternative models based on observational grounds and more such tools need to be developed for bias-free observational data analysis of the Universe.
These findings are described in the article entitled correlcalc: A ‘generic’ recipe for calculation of two-point correlation function, recently published in the journal Astronomy and Computing.