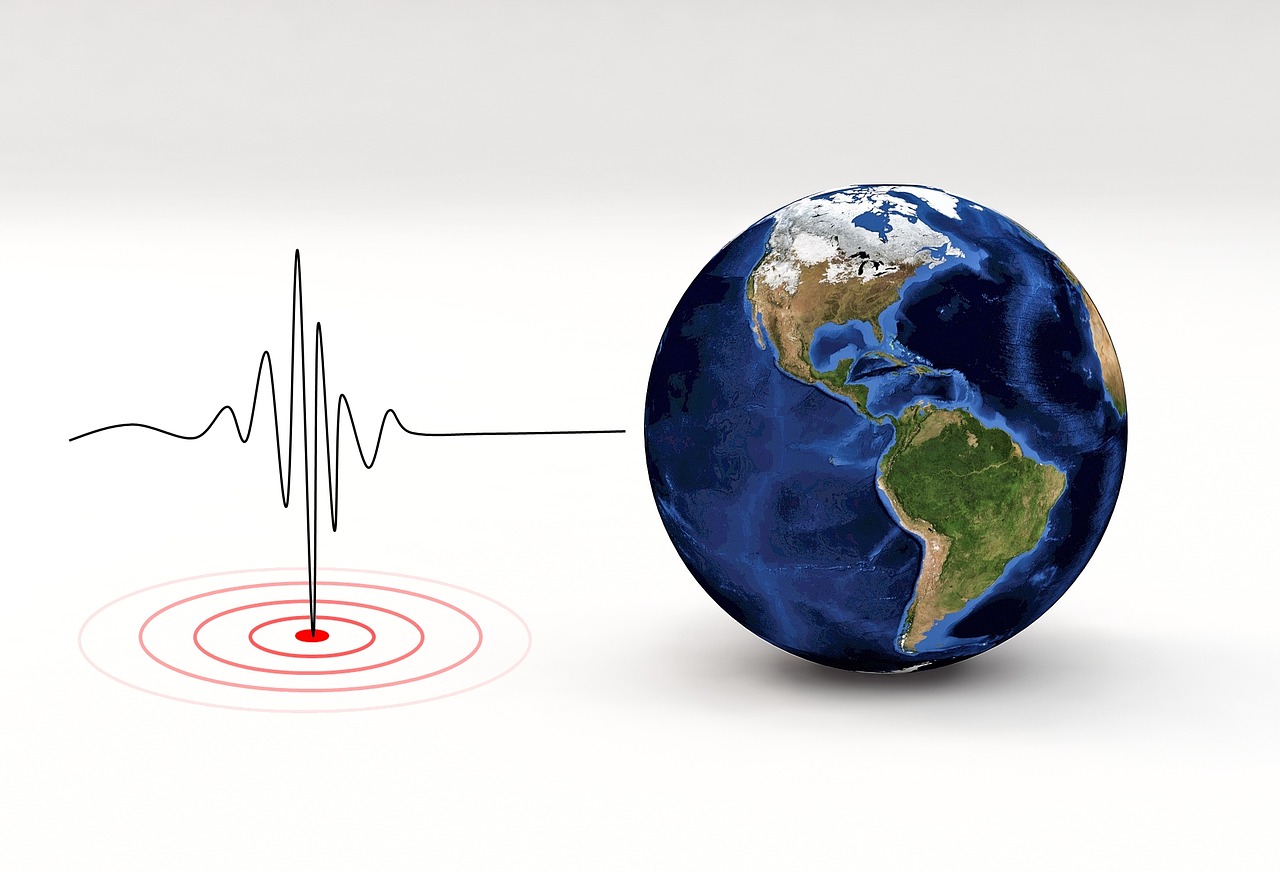
During many natural phenomena, trapped pockets of air or fluid inside the earth’s crust can reach high pressures. For example, geysers occur due to water heating up under the effect of a geothermal energy source. The very high pressure of water vapor created by this process will push its way to reach the surface.
During this movement, the forces exerted between air and solid phases can create deformations and sometimes generate microseismic events. But where do these events come from? How are they organized? What is their relation to the fluid location? How are the fluid channels structured? What are the characteristic properties of this deformation process and of this induced seismicity? How risky are they for the surrounding areas?
To answer these general questions, we have developed an experimental method to study a model system. A fine granular porous medium — for instance, sand — is sandwiched between two glass plates, allowing us to optically observe deformations while fracturing using air injection. A typical pattern is shown in Figure 1.
Compared to natural or industrial situations, the process is faster, happens at lower pressures, and on smaller spatial sizes in our reduced model – it is a downscaled version of an eruption related to an overpressure. Some features are universal: pressurized fluid can lead to branched pathways of large pores and easy flow, and induced seismicity is triggered, both along these pathways and around them.
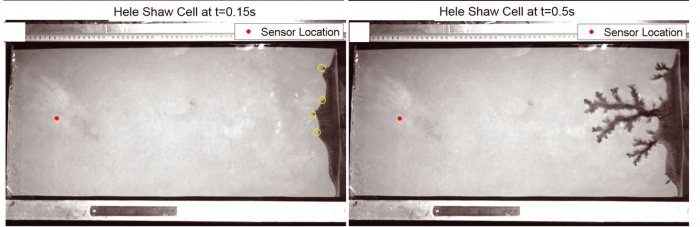
Figure 1. Hele-Shaw cell snapshots during air injection. The cell is 80 cm by 35 cm, and 1 mm thick. It is lying horizontally. The air compacts the porous medium and starts fracturing in several points (yellow circles on left). The fractures advance and get more branches with continuous injection (from the right border, starting at time 0, and maintained at a constant overpressure of 1.5 atmospheric pressure). The red dot shows the location of an accelerometric sensor placed under the cell. Figure republished with permission
The arising patterns depend on the imposed injection pressure level, grain size, humidity, porosity, and injection rate. Here we focus on the fracture-like patterns, which happen at sufficiently large injection pressure (1-2 bars).
Toward the end of the compaction process, the geometry stabilizes, and avalanches rearranging the medium happen in an intermittent way, with an exponentially decaying rate. These events are observed to correspond to a stick-slip behavior (i.e. an interrupted motion rather than a smooth uninterrupted motion) of the large air channel tips.
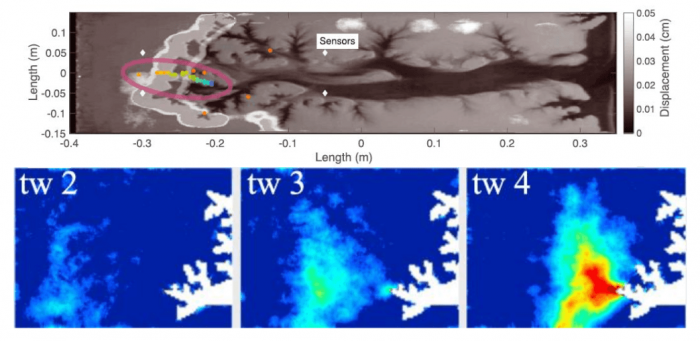
Figure 2. (Top) Results of the microseismic signal localization during fracturing experiments. The color-coded dots from orange to blue shows how the signal source is advancing in time from the porous media towards the channel tips. (Bottom) Image processing results (each frame corresponds to 1 ms) show the deformation field on a close up (warm colors show higher deformation). Figure republished with permission
In this situation, microseismic events are happening away from the main channels (see Figure 2). One of the mechanisms is that the air overpressure diffuses inside the porous medium. This lowers the force exerted through the solid contacts between the grains and plates and leads weak zones to slide and rearrange to a more compact and thus more stable state. These results show that the intuition “deformation starts at the fingertips” during these stick-slip events is not entirely correct. Here, we discovered a pre-development phase where the deformation starts, which corresponds to a slip pulse ahead of the main channel and makes the fingers advance.