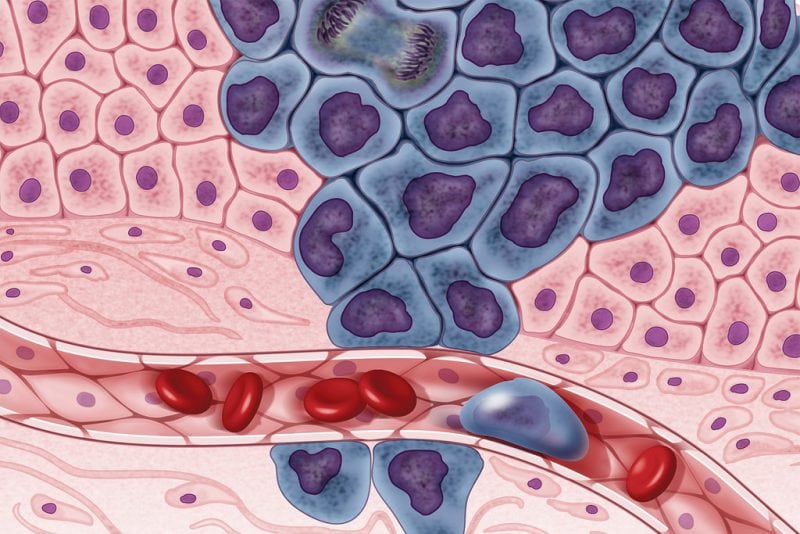
Tumors result from the expansion of cells that bypass the regulatory mechanisms that otherwise control the birth, death, and elimination of cells within healthy tissues. The overarching goal of cancer therapy is to halt such proliferation and eliminate cancer cells while sparing their non-cancerous counterparts. Although many treatments are successful in eradicating a large fraction of cells within tumors, incomplete elimination and the emergence of a resistant population of tumor cells are major hurdles for the success of cancer therapy.
Resistant cells can arise during therapy, or exist in a small fraction of the population, even before treatment. Resistant cells that survive are insensitive to the original treatment and their expansion repopulates tumors leading to relapse in patients. Therefore, rare cells that represent a small fraction of a tumor can play a big role in determining whether a treatment succeeds or fails.
Both genetic and non-genetic mechanisms contribute to the emergence of resistant states
One way in which resistance arises is through genetic changes, i.e. modifications in coding and regulatory DNA sequences (mutations) that impact the type and amount of RNAs and proteins present in the cell. For example, a cell can become resistant to treatment by producing a mutated protein that is no longer affected and inhibited by a particular drug.
In addition, non-genetic mechanisms, which encompass changes in the cellular environment without involving the modification of DNA sequences, are increasingly recognized as drivers of resistance to cancer therapy. The mechanisms underlying non-genetic resistance are quite diverse. Random chance events in production and degradation of RNAs and proteins contribute to variability between cells by leading to different amounts of these molecules. Moreover, cells can differ in how accessible DNA is to the machinery that recognizes specific coding sequences, leading to changes in the genes that are on or off at any given time.
As a consequence, genetically identical cells in a tumor can be in different functional states; for instance, some cells can be dividing while others are in a dormant state. In some cases, these differences between individual cells, or heterogeneity, can translate into cell-to-cell differences in sensitivity to specific treatments.
Distinct mechanisms that generate cell-to-cell variation within tumors, both genetic and non-genetic, may be equally as effective in mediating survival during the initial response to therapy. Specifically, they may lead to similar proportions of cells that are sensitive to a drug. However, the mechanism underlying heterogeneity can profoundly impact the way resistant states change during the course of treatment.
Resistant states differ in how stable they are over time
Genetic changes are generally highly stable, as cells can reliably copy and transfer them to their descendants. Although non-genetic variability can be inherited from mother to daughter cells after cell division, it is usually more easily reversible. Interestingly, the rate at which a non-genetic resistant state reverts to a sensitive one is influenced by the mechanism that drives heterogeneity in the first place.
For instance, cells that are resistant to a drug because they have sporadically produced a large amount of a particular protein regain sensitivity when the levels of such protein return to the average. This can vary between hours to days depending on the lifetime of the specific protein.
At the other extreme, modifications in DNA accessibility generate long-lived resistant states, which can be reliably inherited over many cell divisions. Interestingly, recent research suggests that transient resistant states precede and facilitate the establishment of long-lived ones, or can even become stabilized upon chronic treatment. Understanding the way transient resistant states become progressively more stable is fundamental to maintain sensitivity to treatments for longer time periods.
New technologies are pushing forward our ability to identify and understand the dynamics of rare resistant states
Advances in microscopy have empowered researchers to directly visualize cancer cells as they divide, arrest and die in real time. Combined with our ability to engineer cells to express fluorescent versions of specific signaling molecules, live-cell imaging is shedding light into the molecular basis of heterogeneity in behaviors before and after treatment. Although live-cell imaging provides direct access to the dynamics of proteins within cells, it is limited by the number of signaling molecules that one can simultaneously monitor.
As a complementary strategy, recent technological developments are allowing researchers to quantify the levels of thousands of signaling molecules in each individual cell at a specific time. Such high-resolution “snapshot” is transforming our understanding of the diversity of single cells in healthy and cancerous tissues. It will also provide a platform for the identification of relevant subpopulations of cells to study and further follow through detailed approaches such as live-cell imaging.
Can we keep up with a moving target?
Two properties of tumors challenge our ability to effectively treat cancer. First, individual cells within a tumor vary in their sensitivity to treatments. Rather than being a single target, a tumor is composed of a multitude of targets. Second, cells can switch in and out of resistant states. Therefore, rather than being static, cancer cells are these are moving targets.
A fundamental understanding of the diversity and dynamics of individual cells within a tumor has the potential to alleviate or even exploit these properties in order to prevent the establishment, stabilization, and expansion of resistant cells, enhancing the likelihood of success of existing cancer therapy.
These findings are described in the article entitled Leveraging and coping with uncertainty in the response of single cells to therapy, recently published in the journal Current Opinion in Biotechnology. This work was conducted by José Reyes and Galit Lahav from Harvard Medical School.
Galit Lahav is a Professor and Chair of the Department of Systems Biology in Harvard Medical School. José Reyes is a post-doctoral fellow in Galit Lahav’s lab in Harvard Medical School. Their work advances our fundamental understanding of the causes and consequences of heterogeneity in the response of human cells to DNA damage, in the context of cancer radiotherapy and chemotherapy.