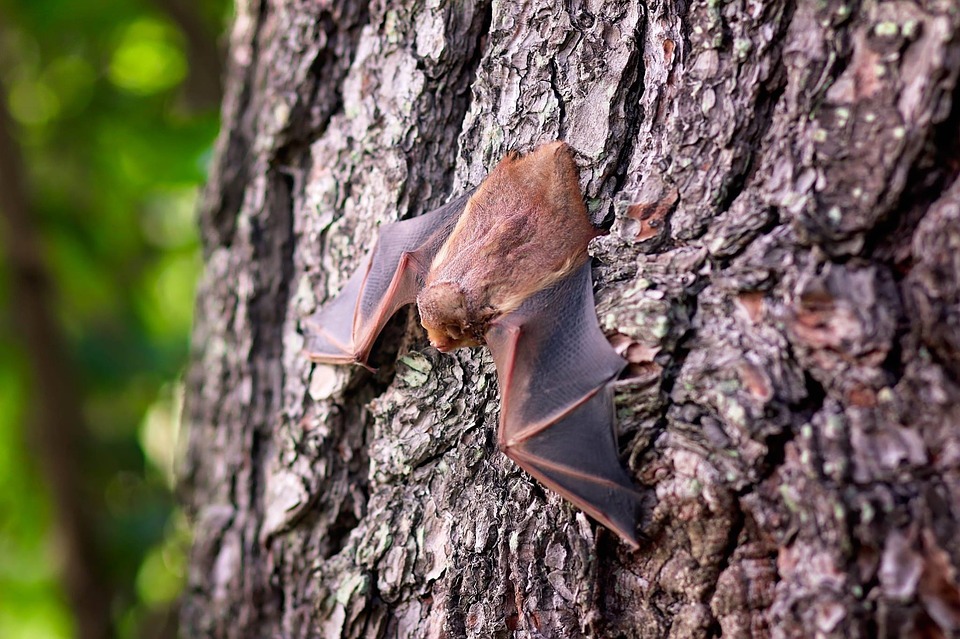
On summer evenings, before it gets completely dark, you may have spotted some flying creatures overhead. Although these creatures could be swallows or other birds out at dusk, there is a good chance that they are bats, the only mammals capable of powered flight. Worldwide, there are >1,300 species of bats, ranging in size from a mere 2 grams to >1 kilogram (Fenton and Simmons, 2014). Of the many features that distinguish bats from other mammals, their use of echolocation has captured the imagination of scientists and the general public alike.
Echolocating animals actively interrogate their surroundings by producing intense, high-frequency sounds and listening to echoes reflecting from objects, see Figure 1 (Griffin, 1958). Echolocation enables bats to extract rich information about objects in the environment, such as the size, direction, and distance of insect prey. Although many insect prey, such as mosquitoes, are only millimeters in size, bats can use echolocation to localize, track, and catch them with ease. Echolocating bats compute the distance to prey by analyzing the elapsed time between emitted calls and echo returns, and they can discriminate target range differences of less than a centimeter. This suggests that bats can determine echo delay with a precision of 60 microseconds or higher. Indeed, over the past few decades, scientists have demonstrated microsecond precision of echo delay measurement in several species of bats (Moss and Schnitzler, 1995; Simmons, 1973). Yet, the big puzzle remains: how can the bat’s brain compute echo delay with such high precision?
One key structure in the auditory system that appears to play a role in the temporal analysis of sound is the inferior colliculus (IC), a midbrain structure found in all mammals. Leading bat neurophysiologists, such as Nobuo Suga and James A. Simmons, have all emphasized the potential importance of the IC in echolocation tasks (Simmons, 2017; Suga, 2015). Following this line of reasoning, Luo and colleagues (2018) investigated temporal processing of sound in the IC of the big brown bat, Eptesicus fuscus (Luo et al., 2018). Specifically, they focused their analyses on the timing of neural responses to echolocation sounds. Rather than measure the timing of action potentials of single neurons, they investigated the precision of extracellular field potentials (EFP’s) that arise from the synchronized activity of populations of neurons. They were surprised to discover that EFP’s exhibited remarkable temporal precision to simulated echolocation calls (Figure 1).
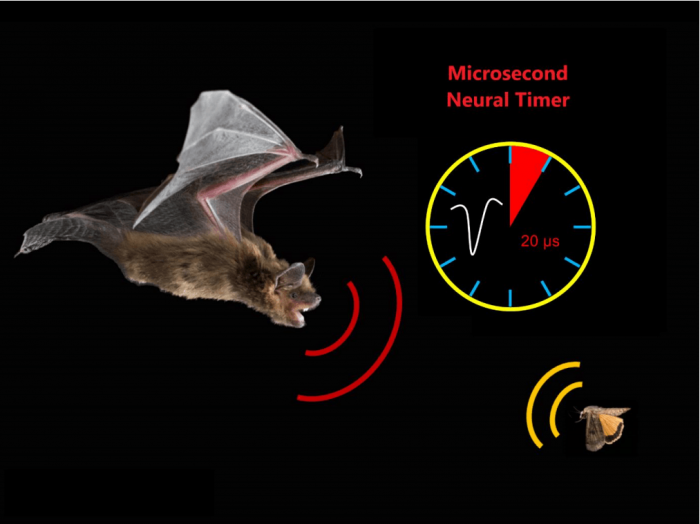
Figure 1: This image shows the big brown bat, Eptesicus fuscus, using echolocation to measure the distance to insect prey. This task requires the brain to compute the time difference between the emitted call and the echo return with microsecond precision. A neural timer with a precision of ~20 µs operates in the auditory midbrain of the bat. Image courtesy Jinhong Luo.
To mechanistically understand how these high-precision EFP’s were generated, Jinhong Luo and Cynthia F. Moss established collaborations with a group of computational neuroscientists, Torbjørn V. Ness and Gaute T. Einevoll at the Norwegian University of Life Sciences and Kechen Zhang at the Johns Hopkins University. Through mathematical modeling, the researchers showed that EFP’s in the bat IC arose from the synchronous firing of a population of neurons, and, importantly, the temporal precision of the EFP’s increased with an increase in the number of firing neurons.
To further explore the functional significance of EFP’s in bat echolocation, the researchers systematically varied the sound parameters of the echolocation calls, including the sound duration and the frequency bandwidth. They found that the highest temporal precision of EFP’s was evoked by echolocation calls of the shortest sound duration and the broadest bandwidth, characteristic of echolocation calls emitted by bats tracking prey. Lastly, the researchers provided further evidence that the high precision EFP’s can accurately estimate the time elapsed between a pair of sounds that simulate a sonar call and echo return, the acoustic cue for sonar target distance measurement. Thus, synchronous firing of a population of neurons can mediate microsecond precision of sound analysis in echolocation tasks, which also bears implications for other types of auditory processing in a wide range of animal species.
These findings are described in the article entitled Neural timing of stimulus events with microsecond precision, recently published in the journal PLOS Biology.
References:
- Fenton, M.B., and Simmons, N. (2014). Bats: a world of science and mystery (University of Chicago Press).
Griffin, D. (1958). Listening in the dark: the acoustic orientation of bats and men (New Haven: Yale University Press). - Luo, J., Macias, S., Ness, T.V., Einevoll, G.T., Zhang, K., and Moss, C.F. (2018). Neural timing of stimulus events with microsecond precision. PLoS Biol. 16, e2006422.
- Moss, C.F., and Schnitzler, H.-U. (1995). Behavioral studies of auditory information processing. In Hearing by Bats, A.N. Popper, and R.R. Fay, eds. (New York: Springer-Verlag), pp. 87–145.
- Simmons, J.A. (1973). The resolution of target range by echolocating bats. J. Acoust. Soc. Am. 54, 157–173.
- Simmons, J.A. (2017). Theories about target ranging in bat sonar. Acoust. Today 13, 43–51.
- Suga, N. (2015). Neural processing of auditory signals in the time domain: Delay-tuned coincidence detectors in the mustached bat. Hear. Res. 324, 19–36.