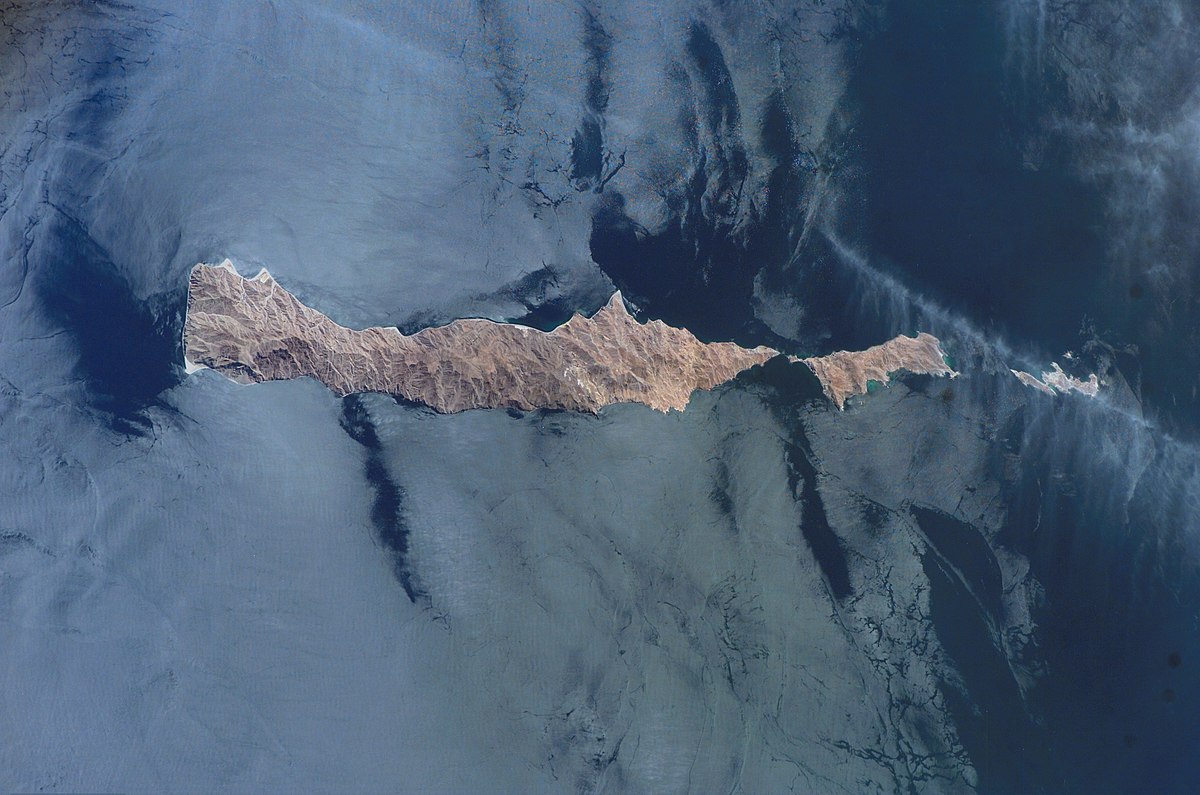
Our entrance into a period of decline in biodiversity is largely recognized, and there is an urgent need to invest more in conservation policies. Because funds allocated to conservation are limited, an essential step is to select sites that, through their management, will preserve the legacy of a region or ecosystem. A good understanding and evaluation of biodiversity are therefore necessary.
Although the efficiency of conservation will finally depend on good governance, through the involvement of all stakeholders and the consideration of socio-economic aspects, various ecological criteria can be used to select sites to protect. The conservation of diversity hotspots, i.e. the spots hosting high diversity levels, can be viewed as “Noah’s arks” or refuge zones to preserve most of the diversity on earth. The number of endemic species is also an important factor because it represents the uniqueness of a site. Then, the maintenance of goods and services for human activity is crucial for effective protection; people need to see the benefits of conservation. Spots with important resources, such as the abundance or biomass of fish, can sustain human activities by allowing highly productive spots to supply the surrounding exploitable areas.
For a few years, another aspect that has been considered is the diversity of biological traits within a community. The diversity of these traits is thought to represent what species do in their environment and how they do it, i.e. the functional diversity of the assemblage. Functional diversity has been shown to highlight new diversity hotspots, to be more sensitive to disturbance and to better evaluate the resilience of assemblages (Stuart-Smith et al. 2013; D’agata et al. 2014; Mouillot et al. 2014).
For the last decade, the number of studies dedicated to functional diversity has soared, particularly for reef fishes. The most common method is to classify reef fish species according to six categorical traits that describe key facets of their ecology and that, in turn, play important roles in ecosystems through regulation of food web and nutrient cycling (Mouillot et al. 2014), i.e. body size, diet, mobility, period of the day at which the fish is active (feeding), level in the water column, and gregariousness (Figure 1). This functional trait matrix can be transformed into a dissimilarity matrix among species (how dissimilar species are compared to each other), from which we can get the principal coordinates scores of each species (thanks to a Principal Coordinate Analysis). In other words, each species can be positioned in a functional space which represents the best interests of the method because it allows us to quantify a distance separating each species according to its ecological traits (Figure 1).
From these distances, we can study various facets of biodiversity, including the functional richness, i.e. the functional volume encompassing all the species; the functional complementarity, i.e. how different are the species to each other; or the functional redundancy, i.e. how many species occupy a similar position in the functional space (see Mouillot et al. 2013). In our study, we propose to use functional divergence among fish assemblages of different regions to target sites of interest for conservation. We define functional divergence between assemblages to be when these assemblages are dominated by different species characterized by different functional traits (occupying a different position in the functional space). Conversely, a functional convergence occurs when distinct species compositions share the same functional traits. Such a functional divergence could highlight the importance of some regions, although they are not recognized as diversity hotspots or to host a high number of endemic species (Figure 1).
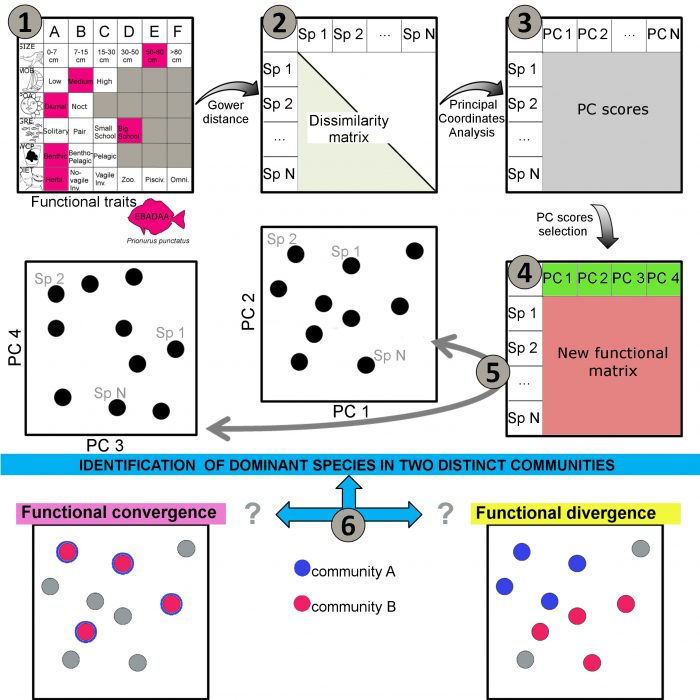
Figure 1. Methods used to identify functional divergence between reef fishes assemblages. 1) An ecological trait matrix is built. 2) A dissimilarity matrix (with gower distance) is obtained from the functional matrix. 3) A principal component analysis is performed to get the PC scores of each species in N dimensions. 4) A selection of the number of PC axes is made (see Maire et al. 2015). 5) Each species is positioned according to its coordinates in a functional space (4 dimensions here). 6) The dominant species in each assemblage are identified and positioned in the functional space. Figure courtesy Damien Olivier.
The Gulf of California (GC, Mexico) is among the most biologically rich marine regions on Earth. The GC is a dynamic, semi-closed marginal sea of the Eastern Pacific (approximately 1000 km long and 150 km wide) with important latitudinal oceanographic and biogeographic variation, e.g. seasonal temperatures, tidal mixing, bathymetry, and geomorphologic diversity. One specific aspect of the GC seascape is the presence of a patchwork of ~80 large islands and ~800 islets, designated as a UNESCO World Heritage Site. The seas and rocky reefs around all of these islands are essential refuges for the region’s littoral and shallow-water biodiversity. At the same time, the GC is of high economic value for the fishing industry, accounting for 70% of the catch in Mexico. Although the human population density is relatively low in the region, it is rapidly increasing, and the GC has not been exempt from coastal and marine degradation trends.
Most of the major islands in the GC belong to two distinct regions, i.e., the “Midriff Islands” and the “Loreto-Cerralvo Corridor” (LC Corridor) in the northern and southwestern part of the GC, respectively (Figure 2). These two set of islands represent potential MPA clusters for the GC. Our main results highlight that the LC Corridor hosts 94% of the reef fishes species and 98% of the functional richness, while the Midriff Islands represent 63% of the species and 83% of the functional richness. A priori, the conservation of the LC corridor will offer an important protection level for the reef fishes of the GC. However, if we go further and identify which species dominate the biomass of the assemblages in both regions, we notice that the fish biomass in both regions is dominated by different species characterized by different ecological-traits, i.e. occupying different positions in the functional space (functional divergence) (Figure 2).
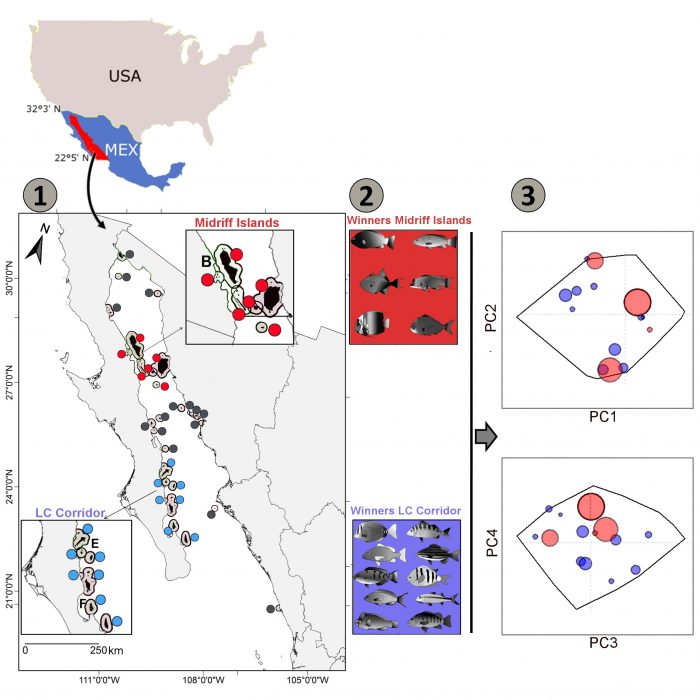
Figure 2. 1) Distributions of survey locations in the Gulf of California. In red, are highlighted the sites belonging to the Midriff Islands and in blue are highlighted the sites of the LC Corridor. 2) Identification of the winner species (those favored in one or another of the two regions). 3) The positioning of the winner species of both regions in the functional space. The red dots correspond to the winner of the Midriff Islands and the blue ones to those of the LC Corridor. The size of the circle is proportional to the gain of the species, i.e. how more important is a species in one region in comparison to the other. Figure courtesy Damien Olivier.
In the north, large solitary benthic invertivores such as triggerfish, wrasse, or sea bream are favored, while this is not true for the LC corridor. In the south, large solitary herbivores such as parrotfishes and highly gregarious zooplanktivores such damselfishes and serranids are favored. Furthermore, the piscivores are dominated by a solitary grouper in the north but by highly gregarious species such as snappers and grunts in the south.
We concluded that although the north region is not identified as a diversity hotspot, the functional divergence shows that both regions are complementary to each other because a Marine Protected Area including the Midriff Islands should better preserve some functions, while the protection of additional functions would be more efficient within another MPA in the LC corridor. The functional divergence between northern and southern regions may be explained by the high gradient of oceanographic variables along the GC which should be considered as two separated entities (with proper conservation policy) instead of a single homogenous sea.
These findings are described in the article entitled Functional-biogeography of the reef fishes of the islands of the Gulf of California: Integrating functional divergence into marine conservation, recently published in the journal Global Ecology and Conservation.
References:
- D’agata, S., D. Mouillot, M. Kulbicki, S. Andréfouët, D. R. Bellwood, J. E. Cinner, P. F. Cowman, et al. 2014. Human-Mediated Loss of Phylogenetic and Functional Diversity in Coral Reef Fishes. Current Biology 24:555–560.
- Maire, E., G. Grenouillet, S. Brosse, and S. Villéger. 2015. How many dimensions are needed to accurately assess functional diversity? A pragmatic approach for assessing the quality of functional spaces. Global Ecology and Biogeography 24:728–740.
- Mouillot, D., N. A. J. Graham, S. Villéger, N. W. H. Mason, and D. R. Bellwood. 2013. A functional approach reveals community responses to disturbances. Trends in ecology & evolution 28:167–177.
- Mouillot, D., S. Villéger, V. Parravicini, M. Kulbicki, J. E. Arias-González, M. Bender, P. Chabanet, et al. 2014. Functional over-redundancy and high functional vulnerability in global fish faunas on tropical reefs. Proceedings of the National Academy of Sciences of the United States of America 111:13757–62.
- Stuart-Smith, R. D., A. E. Bates, J. S. Lefcheck, J. E. Duffy, S. C. Baker, R. J. Thomson, J. F. Stuart-Smith, et al. 2013. Integrating abundance and functional traits reveals new global hotspots of fish diversity. Nature 501:539–42.