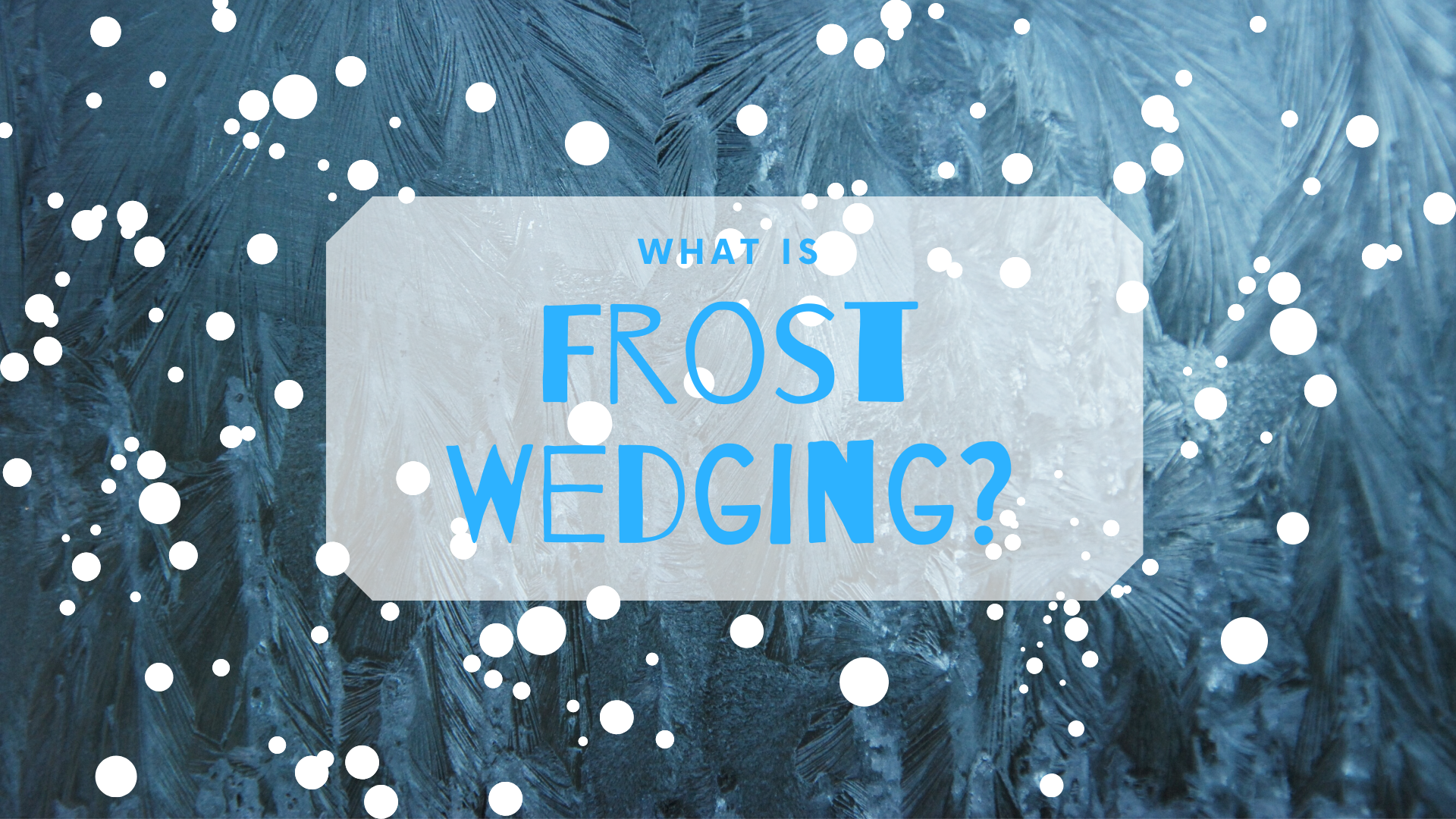
Frost wedging is the process by which water/ ice weathers and erodes landscapes through repeated freeze and thaw cycles. As water freezes it expands by 9 percent, wedging the rock apart only to melt again during the summer months.
The geomorphology of subarctic environments is greatly influenced by processes associated with cycles of freezing and thawing. A single freeze-thaw cycle may be many years in length, or it may be a seasonal or diurnal occurrence. Both the number of cycles and their intensity (i.e. temperature range) are important factors when determining the causes of physical weathering, a general term which encompasses in part the processes of frost cracking, frost wedging, frost heaving, and frost sorting, each of which will be briefly discussed here.
Many other environmental factors also influence the efficacy of frost action, such as the presence or lack of permafrost and tree cover, the type of soil or bedrock in question, and especially the variability of climate and topography. Here we will begin with an overview of geographical and climatic information about subarctic environments, followed by a description of freeze-thaw cycles and some frost action processes. Finally, some periglacial landforms, such as patterned ground, will be presented. This article will not discuss the processes and resultant landforms of mass-wasting associated with freeze-thaw cycles.
Frost Wedging In Cold Environments
In North America, subarctic environments are found in northern Canada and Alaska; elsewhere they are found in northern Europe and Asia. These regions exhibit “strong seasonal variation [in temperature] with short, moderately warm and moist summers and long, extremely cold and dry winters.” There are, however, exceptions to this generalization, the most obvious of which are climatic variations due to changes in topography.
“All that is gold does not glitter, Not all those who wander are lost; The old that is strong does not wither, Deep roots are not reached by the frost.” — J. R. R. Tolkein
In addition, relative proximity to oceans has the effect of minimizing the seasonal range of temperatures, for example in coastal regions of Canada and Scandinavia. In more continental locations, such as the interiors of Alaska or Russia, yearly maximum, and minimum temperatures can vary by more than 100 degrees Celsius. Precipitation also varies considerably among different subarctic regions. In eastern Canada, annual precipitation reaches 81 cm in some places, whereas in areas of Alaska where mountain ranges obstruct the movement of moist air, annual precipitation may be as low as 18 cm.
Local variations in temperature and precipitation are two factors that influence the processes of frost action, as will be explained below. Additional environmental factors are snow and forest cover, both of which tend to regulate the temperature of soil and bedrock. In summer months, forested ground (being cooler than exposed ground), thaws less quickly and deeply, while in winter months it remains warmer than the exposed ground. The ground which is covered by extensive snowfall or ice cover is somewhat insulated from changes in air temperature.
How Permafrost Impacts Physical Weathering
Another important environmental factor is the presence of permafrost, defined by S.W. Muller as “a thickness of soil or other superficial deposit, or even of bedrock, at a variable depth beneath the surface of the earth in which a temperature below freezing has existed continually for a long time (from two [years] to tens of thousands of years)”. Permafrost is classified as either continuous or discontinuous, the latter implying that its distribution over a landscape is not contiguous.
The soil located above permafrost is called the active layer, and it goes through typical cycles of freezing and thawing. Because permafrost is oftentimes an impermeable layer, it can prevent water from the thawing ice and snow above it from percolating deeply and becoming runoff, which keeps moisture in the soil, making it more prone to the effects of frost action. In other circumstances, the decreased permeability of frozen ground may also lead to more runoff and thus greater risk of flooding.
Some dependent factors which influence a landscape’s susceptibility to frost action are bedrock and soil structure, mineral composition, grain size and the impact of human activities. These are variables that, among other things, affect the thermal conductivity of the ground. For example, a cycle of freezing and thawing will affect sandy clay at much greater depths than it will affect dry sand.
Ice Lenses & Frost Wedging
If sufficient moisture is present in soil which undergoes relatively slow freezing, it is likely to segregate into ice lenses, which are typically horizontal layers of ice parallel to the freezing front. Water contributing to the growth of ice lenses may come from the pores of rock and soil, or it may be supplied from sources such as snowmelt, permafrost melting, and surface or groundwater. Ice lenses range in size from a few millimeters to many meters, and in forming the separate sections of soil and tend to move it in a vertical direction, i.e. perpendicular to the freezing front.
More often than not, ice lenses form a “series” composed of numerous small lenses, rather than in a single large lens. It is thought that “an electric double layer adjacent to a mineral surface, and the dipole nature of water are basic elements in the movement of water to a freezing plane,” although researchers still lack an exact explanation. Regardless of the cause, it seems that soil moisture which undergoes freezing is affected by a segregation “suction potential,” which increases or decreases according to variables such as grain size and mineralogy. In general, soils with finer grain size accumulate of larger ice lenses and are thus more susceptible to frost heaving; soils with extremely fine grain size, however, may become impermeable to the movement of water towards a freezing front, in which case ice lenses will not form.
As described above, the segregation of ice can occur when the rate of freezing and grain size are conducive to this process. In cases of very rapid freezing, however, pore water is essentially sealed off and is unable to migrate towards a freezing front.
“Like frost, beautiful things are being born in us.” — Joyce Rupp
The growth of ice lenses contributes to the phenomenon of frost heaving, which is the upward movement of soil during freezing. It is thought that upfreezing, which occurs when a freezing front moves from the permafrost upwards, is responsible for the heaving of buried rocks and even joint blocks to the surface over the course of many freeze-thaw cycles. Two different hypotheses, termed “frost-pull” and “frost-push,” have been proposed to explain the heaving of buried objects.
It is interesting that objects which are oriented vertically, i.e. perpendicular to the freezing front, experience a greater degree of heaving than those which are oriented parallel to the freezing front; moreover upfreezing has the tendency to rearrange objects such that they are oriented vertically. Furthermore, larger objects have a tendency to experience a greater amount of heaving that smaller ones.
In addition to grain size and temperature, the most important factors which determine the degree of frost heaving are soil moisture, the presence of vegetation, and soil depth. Research indicates that heaving is greater in areas that are wetter and more sparsely vegetated. Also, studies have shown that heaving in the active layer increases with depth.
Frost wedging is the name given to the prying apart of rock due to the expansion of freezing water. This process is especially effective when moisture content is high and freezing is rapid; in such cases, water in pores and cracks is sealed off and unable to migrate, creating internal stresses that are sufficient to shatter the rock. Susceptibility to frost wedging depends largely on lithology. Sedimentary rocks tend to shatter more easily than igneous rocks, and rocks with larger pore sizes are more susceptible than those with smaller pore sizes. It should also be noted that some research indicates that the degree of frost wedging is proportional to the intensity, number, and length of freeze-thaw cycles a rock undergoes.
Another important aspect of frost action is the formation of needle ice, a process that occurs at or just below the ground’s surface and is characterized by the growth of long filaments of ice in a vertical direction. This can have the effect of displacing the topmost layer of soil, sometimes up to 40 cm. Needle ice is more prominent in soil that is not vegetated, and when it accompanies multiple cycles of freezing and thawing, its tendency to displace sediment vertically leads to a greater susceptibility to wind action and turf deflation.
The surface evidence of frost action varies according to the lithology and topography of a given environment; needless to say, the type and intensity of frost action involved are also important in determining surface forms. On a small scale, it is common for stones lying on the ground’s surface to be surrounded by slight gaps in the soil. These gaps are evidence of frost heaving.
“It is the life of the crystal, the architect of the flake, the fire of the frost, the soul of the sunbeam. The crisp winter air is full of it.” — John Burroughs
Additional surface evidence of frost action is the existence patterned ground, “a group term for the more or less symmetrical forms, such circles, polygons, nets, steps, and stripes, that are characteristic of, but not necessarily confined to, mantle subject to intensive frost action.” For descriptive purposes, patterned ground is categorized as either sorted or unsorted. A sorted patterned ground is characterized by a separation of stones and finer sediment into one of the various geometric forms listed above. The size and orientation of patterns are influenced by factors such as the orientation of the freezing front, the freezing rate, soil moisture, and grain size, density and shape. Frost sorting is accomplished by several different types of frost action, including upfreezing (heaving), displacement by needle ice, and mass displacement.
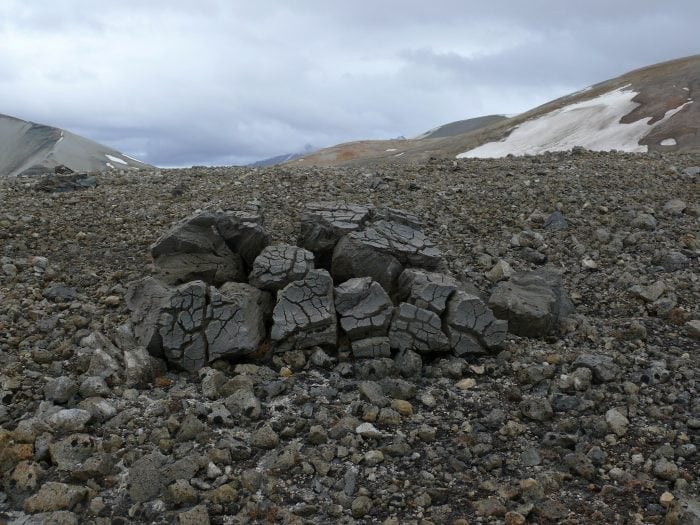
Frost Wedging. “Breadcrust bomb near Novarupta, Valley of Ten Thousand Smokes, Memorial Day Weekend 2010 079” by Katmai National Park and Preserve via Flickr is licensed under CC0
Frost wedging is another process that produces recognizable surface forms. As described above, when water in the cracks or pores of a rock freezes quickly, the internal stresses acting on the rock can be sufficient to shatter it. Over landscapes where frost wedging is a dominant process, rocks are typically angular and of greatly varying size. In areas where frost sorting is taking place, the fragments of rock may be patterned according to size.
In addition to creating unique surface orientations of rocks, frost action processes are thought to influence other surface processes, such as erosion. The effect of freeze-thaw cycles on rill sediment transport rates on Prince Edward Island was studied by Edwards, Burney, and Frame (1995). The area studied was primarily agricultural, so experimental conditions were designed to mimic those of a large, tilled field. It was found that soil that had undergone several freeze-thaw cycles produced 25% more sediment yield during experimental trials than did soil under similar conditions, but unfrozen.