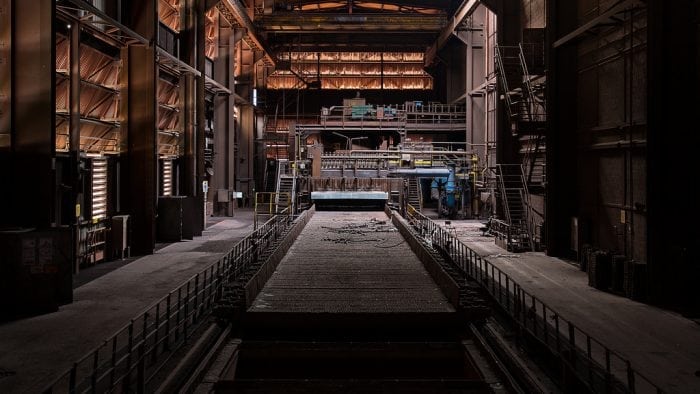
Manufactured ceramics are one of the first technological achievements of humankind, and after thousands of years, although their spectrum of applications extends far beyond pottery ware, processing control is still a major challenge, in particular, if the goal is to achieve pore-free materials, a necessary condition for optical applications (such as lasers and armored windows, for instance).
A critical and (in most cases) the limiting step in processing fully dense parts is the so-called sintering, which is the technical name for heating up to high temperatures so that ceramics becomes a strong-cohesive part. Explaining a bit more; different from metals that can be typically melted or deformed for molding, ceramics start as a powder, such as in clays, that although can be mixed with water for molding, needs to be heated up so that the particles combine and pores are eliminated. The driving force for sintering is the same as when one combined two droplets of water so that they spontaneously become a bigger single droplet: the surface tension. Because of the high melting points of ceramics, very high temperatures are needed, not to melt, but to give enough heat so that atoms from two particles can have enough mobility so they bind from the surface and pores are eliminated (imagine three ice cubes touching forming a triangle: at room temperature: they bind to each other and then the void in between slowly disappears).
In ceramics, the problem is that depending on the composition, eliminating pores can be very difficult. This leads to major technological challenges that are still solved on a purely empirical basis. Theories dated of 1950’s attempt to explain why this is so by suggesting mass transport mechanisms are different depending on the composition and temperatures, and some of those mechanisms favor pore elimination, but others don’t. Although those theories are still largely accepted, they contain huge oversimplifications of the problem, some due to a limitation on the understanding of the process, and some due to a lack of computer power to calculate actual solutions for the proposed analytical models. In truth, one of the greatest simplifications assumed there is that the thermodynamic driving force is constant.
That is, surface energy is the driving force, and this number is constant and is not affected by impurities, for instance. Another oversimplification is the overlook of the role of grain boundary energies (which is the new interface formed between two or more crystalline particles during sintering) in controlling the process.
A UC Davis team in collaboration with the NPU, China, has addressed this problem and developed a theoretical framework in which thermodynamics and kinetics of sintering are both varying during sintering and have a strict dependence on their compositions, such that segregation of impurities to both surfaces and grain boundaries have profound effect on the process development. This opens a perspective to understand and enable the design of the impurities as sintering aids to induce full densification and controlling microstructure (e.g. grain size). One of the greatest discovered from the model is that thermodynamics (i.e. the energies of both surface and grain boundary) is way more relevant and dynamic than previously perceived and can hold the key for optimal designing of sintering.
They report a mutual interaction between grain growth and densification which essentially depends on the interlocked driving forces of those processes: the surface energy contributes positively to the driving force of grain growth while the grain boundary energy negatively to the driving force of densification. This suggests a smart strategy to improve densification by selecting impurities (aka dopants) to create favorable thermodynamic scenarios.
These findings are described in the article entitled Modeling the final sintering stage of doped ceramics: mutual interaction between grain growth and densification, published in the Journal of Materials Science.