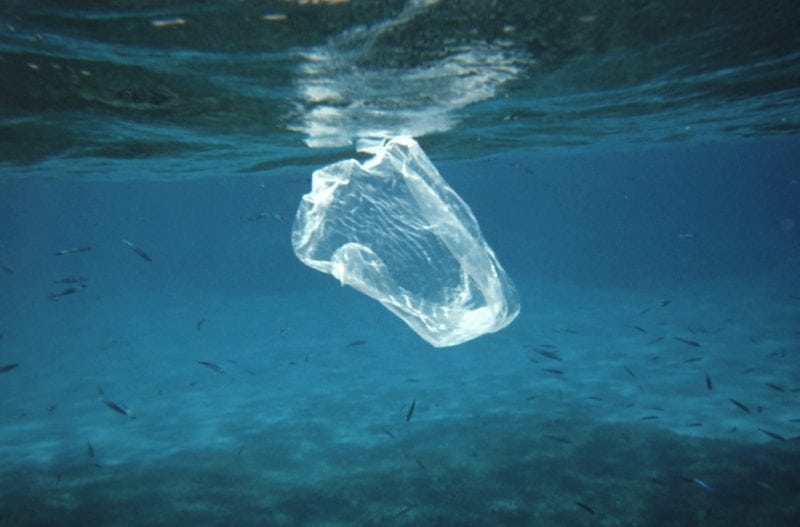
Small particulates that exist in the environment are produced either naturally within groundwater or through anthropogenic activities. Ultra-small particles in micron or smaller size (1 micron = 10-6 m) are of particular interest because they are often found chemically more reactive than the same materials in a larger size. Studying chemistry of a micro- or nano-sized particle will, moreover, help us understand its intrinsic reactivity. This could further lead us to identify some of the environment-specific properties of the solid, such as solubility, catalytic activity, and toxicity.
For example, particles form in groundwater through nucleation of mineral clusters and are an important source of nutrients for microorganisms and plants. With the knowledge of chemical reactivity of naturally-existing particles is a key to understand how mineral ions are released from particles. Furthermore, it is important for us to know the chemistry of the particle surface with pollutants so that we can predict whether pollutants that have been taken up by a mineral particle would be reintroduced elsewhere or permanently eliminated from the groundwater via sedimentation.
Ultra-small particles also come to exist in nature due to anthropogenic activities. Today, there is a mass production of tailored small particles for commercial purposes. These particles often have enhanced chemical reactivity, which could have a detrimental effect on our health and the natural ecosystem once they are leached away.
Ultra-small plastic particles could also be harmful to our health. Specifically, plastic particles may be able to adsorb other chemicals, such as persistent organic pollutants from the surrounding environment, which are then bio-accumulated in our food through ingestion by aquatic organisms. In addition, unlike engineered particles, plastic particles are chemically stable and do not undergo natural degradation. For those reasons, it is important for us to be able to determine the health effects and eco-toxicity of ultra-small particles and to continuously monitor their presence in the natural ecosystem.
A challenge to study the chemical reactivity of small particles is that a single ultra-small particle is too small to be manually attached to any existing analytical probes. In addition, studying them in a large cluster tends to lose some of their intrinsic chemical reactivity and they would not be in the same condition as they are in the environment. In water, all suspended particles moves randomly; this is called the Brownian motion.
So, instead of trying to fix a particle onto a probe, our analysis waits for a particle to randomly collide with the probe. Furthermore, in order to detect the impacting particles, potential is applied to the probing electrode. When an electrode potential is sufficient to observe chemical reaction at, or of, a colliding particle, a transient signal response, which typically looks like a spike, will be recorded the by potentiostat. Figure 1 presents a graphical representation of a particle-impact event and a typical chronoamperogram (time-current curve).
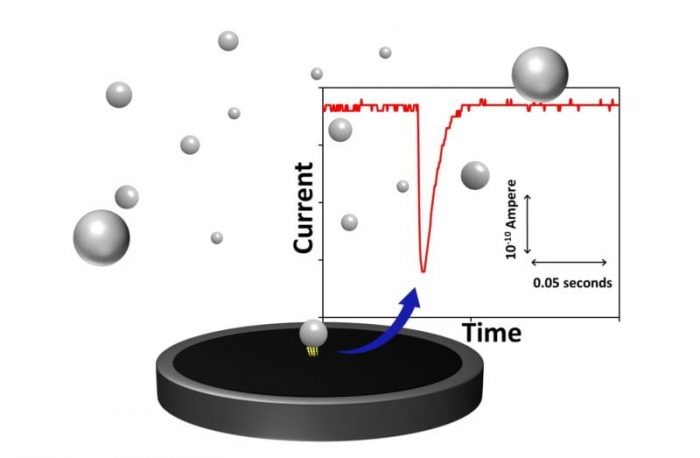
Figure 1. Graphical representation of a single particle undergoing an electrochemical process upon collision with a stationary probing electrode. Transient current response resulting from the electrochemical reaction typically looks like a spike in chronoamperogram, which is also shown in this figure. Credit: Kenichi Shimizu
It is found that the observed current is from the electrochemical reduction of oxygen molecules that has been adsorbed by a plastic particle. Plastic itself cannot be reacted or facilitate any reaction because it is an electrically insulating and chemically non-reactive material. Moreover, once the absorbed oxygen is purged by passing pure nitrogen gas for a prolonged time, no spike is recorded. This may be implied that plastic micro-particles readily adsorb other chemical substances from the environment though further study is needed.
For polyethylene, solubility of oxygen is known, hence we could estimate the particle size based on the amount of oxygen found in each particle, which is obtained from the integrated area under a pike. In this proof-of-concept study, we are able to show that the size distribution of plastic micro-sphere that is derived from the electrochemical analysis is in good agreement with the observation using a scanning electron microscope. This result is highly important because there will be no need of extracting particles from the sample for size determination if the characteristic chemical reaction of the particle can be identified.
It is found that the number of pikes observed per measurement is dependent of the particle concentration. That is, we observed more spikes as the concentration of plastic micro-particles were gradually increased. Furthermore, we are actually able to create a calibration curve, which is a linear correlation between the concentration of plastic particles and the number of observed spikes. It is furthermore encouraging that the calibration curve includes over roughly three orders of magnitude of the particle concentration (from 3.6 × 109 particles per litter to 1.4 × 1011 particles per litter). Our finding suggests that the concentration of small plastic debris in natural aquatic samples may be analytically determined using the particle-impact approach.
In conclusion, ultra-small particles can be studied individually while they are still suspended in water by making use of random collision between a particle and a stationary probing electrode. Furthermore, by applying potential to the electrode, chemistry involving the impacting particle can be studied. This approach is also promising analytical method to determine the particle concentration in its natural aquatic environment.
These findings are described in the article entitled In situ Detection of Microplastics: Single Microparticle-electrode Impacts, recently published in the journal Electroanalysis. This work was conducted by Kenichi Shimizu, Stanislav V. Sokolov, Enno Kätelhön, Jennifer Holter, Neil P. Young, and Richard G. Compton from Oxford University.