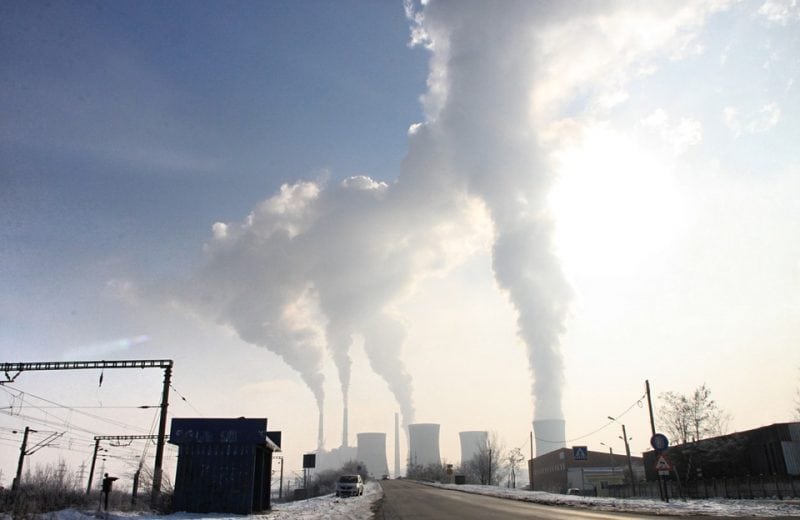
Global warming is a worldwide issue caused by the increasing greenhouse gas emissions due to human-induced activities. In order to control the CO2 emission in the short term, Carbon Capture and Storage (CCS) has been proposed as one of the major strategies that have an immediate effect on the mitigation of global warming [1].
The pre-combustion CO2 capture through an integrated gasification combined cycle (IGCC) is one of the possible approaches to equip fossil fuel power plants with CCS [2].
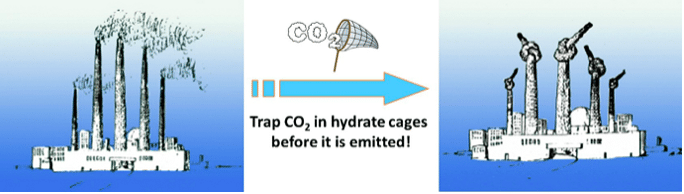
(Republished with permission from Applied Energy)
The typical fuel gas composition coming out from the IGCC process is around 40% CO2 and 60% H2 with its pressure between 2.0 and 7.0 MPa [3]. Hydrate-based gas separation (HBGS) is one of the attractive methods to capture CO2 from fuel gas mixtures [4]. Gas hydrates (also called clathrate hydrates) are ice-like inclusion crystals in which water molecules form the framework through hydrogen bonding and guest molecules of suitable size like CO2, CH4 etc. are trapped inside [5, 6].
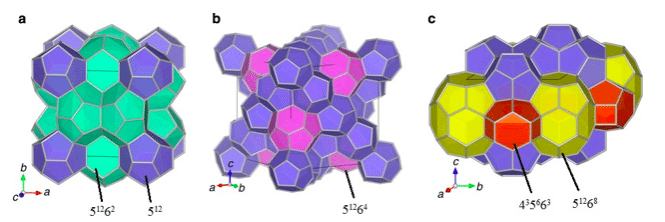
Figure 1. Three typical structures of gas hydrates: (a) sI, (b) sII, and (c) sH. Water molecules form cages. Guest molecules are trapped in those cages. (Republished with permission from Applied Energy)
When a fuel gas mixture (CO2/H2) is subjected to hydrate formation at a high pressure and low temperature, CO2 has more preference over H2 to be trapped in hydrate cages, thus separating CO2 from fuel gas mixture[8]. The schematic of this process is shown in Figure 2. The advantages of this process include the high CO2 storage capacity, high energy efficiency and clean process (predominantly water is used as solvent).
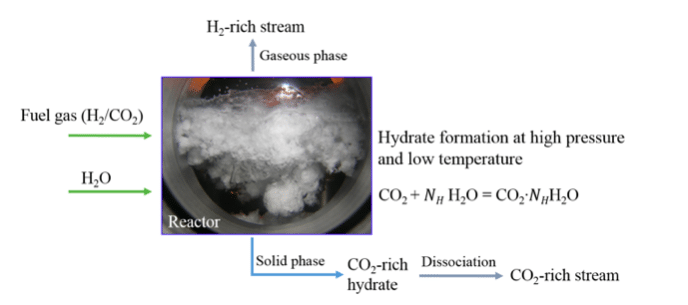
Figure 2. Hydrate-based CO2 capture from fuel gas mixture. (Republished with permission from Applied Energy)
At 6.0 MPa, the equilibrium temperature of hydrate formation from fuel gas is 274.6 K [9]. HBGS process must be operated much below this temperature for a sufficient kinetic driving force to enable it which incurs a significant refrigeration cost for this technology. In order to reduce the refrigeration cost, researchers have identified various thermodynamic promoters such as propane and tetrahydrofuran (THF) to mitigate the equilibrium conditions, as they can occupy the large cages of the hydrate structure and leave the small cages to accommodate the CO2 molecules (refer to Figure 1 for the illustration of large and small cages).
Recently, another promoter, tetra-n-butylammonium fluoride (TBAF), was found to have an excellent thermodynamic promotion effect on hydrate formation [10, 11]. Unlike THF and propane, TBAF forms semiclathrates in which the promoter molecules not only occupy the cages but also constitute a part of the host framework [12]. The equilibrium temperature of CO2/H2 hydrate in the presence of 3.38mol% TBAF is around 302.1 K at 6.0 MPa, which makes the hydrate formation process feasible to be operated at room (or ambient) temperature.
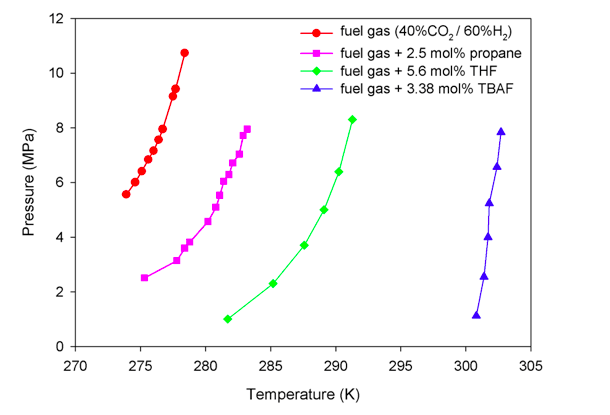
Figure 3. Phase equilibrium curves for hydrate formation from fuel gas [9], and in the presence of 2.5 mol% propane [13], 5.6 mol% THF [14], or 3.38 mol% TBAF [11]. Hydrates can only form at conditions above the phase equilibrium curves. TBAF exhibits the most significant effect on mitigating the equilibrium conditions. (Republished with permission from Applied Energy)
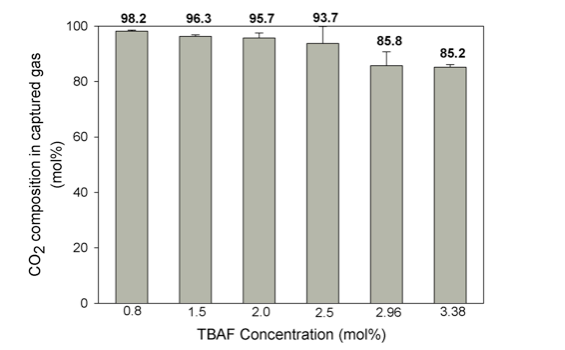
Figure 4. CO2 composition in the captured gas after hydrate formation with various TBAF concentrations. (Republished with permission from Applied Energy)
While the addition of thermodynamic promoters can moderate the hydrate formation conditions, there is another category of promoters known as the kinetic promoters. They can enhance the hydrate formation kinetics without affecting the equilibrium conditions. Further, in this study, we tested the promotion effect of one typical surfactant, sodium dodecyl sulfate (SDS), and two environmentally benign amino acids (leucine and tryptophan) as the kinetic additives.
Compared with the case without kinetic additives, all additives studied in this work were able to enhance the nucleation and initial rate of hydrate formation. Furthermore, a different hydrate morphology was observed with the addition of some kinetic additives. Figure 5a shows the hydrate morphology for the case without kinetic additives, hydrate particles accumulate in a uniform manner; while with the addition of 3000 ppm tryptophan (Figure 5b), we can see an irregularly shaped hydrate chunk in the mushy bulk mixture, and a dendritic structure for the hydrates growing on the window. Such a change in morphology may be related to the hydrophobicity of the amino acids and the interactions between their functional groups and the hydrate particles. Further research including detailed characterization is required to gain more insights into the roles of amino acids.
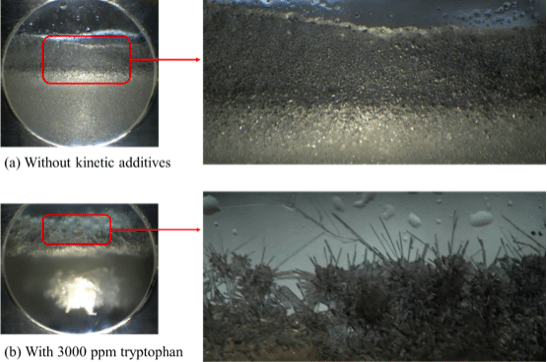
Figure 5. Different morphologies of hydrates formed with and without kinetic additive. (Republished with permission from Applied Energy)
Overall, the high CO2 composition enriched (up to 98.2 mol%) and the ambient operating temperature highlight the advantages of employing TBAF in the hydrate-based CO2 capture process. It is worthwhile to make more efforts to further enhance the kinetics of this process for future application.
These findings are described in the article entitled Semiclathrate based CO2 capture from fuel gas mixture at ambient temperature: Effect of concentrations of tetra-n-butylammonium fluoride (TBAF) and kinetic additives, recently published in the journal Applied Energy. This work was conducted by Junjie Zheng, Krittika Bhatnagar Maninder Khurana, and Praveen Linga from the National University of Singapore, Peng Zhang from Shanghai Jiao Tong University, and Bao-Yong Zhang from Heilongjiang University of Science and Technology.
References
- IEA. Energy technology perspectives 2015: Executive summary. International Energy Agency; 2016.
- Hendriks CA, Blok K, Turkenburg WC. Technology and cost of recovering and storing carbon dioxide from an integrated-gasifier, combined-cycle plant. Energy. 1991;16:1277-93.
- Metz B, Davidson O, Coninck Hd, Loos M, Meyer L. IPCC 2005: IPCC special report on carbon dioxide capture and storage.: Cambridge University Press: Cambridge, United Kingdom and New York, NY, USA; 2005.
- Babu P, Linga P, Kumar R, Englezos P. A review of the hydrate based gas separation (HBGS) process for carbon dioxide pre-combustion capture. Energy. 2015;85:261-79.
- Sloan ED. Fundamental principles and applications of natural gas hydrates. Nature. 2003;426:353-63.
- Englezos P. Clathrate hydrates. Industrial & Engineering Chemistry Research. 1993;32:1251-74.
- Momma K, Ikeda T, Nishikubo K, Takahashi N, Honma C, Takada M, et al. New silica clathrate minerals that are isostructural with natural gas hydrates. Nature communications. 2011;2:196.
- Linga P, Kumar R, Englezos P. The clathrate hydrate process for post and pre-combustion capture of carbon dioxide. Journal of Hazardous Materials. 2007;149:625-9.
- Kumar R, Wu H-j, Englezos P. Incipient hydrate phase equilibrium for gas mixtures containing hydrogen, carbon dioxide and propane. Fluid Phase Equilibria. 2006;244:167-71.
- Zheng J, Zhang P, Linga P. Semiclathrate hydrate process for pre-combustion capture of CO2 at near ambient temperatures. Applied Energy. 2017;194:267-78.
- Park S, Lee S, Lee Y, Seo Y. CO2 capture from simulated fuel gas mixtures using semiclathrate hydrates formed by quaternary ammonium salts. Environmental Science & Technology. 2013;47:7571-7.
- Aladko LS, Dyadin YA, Rodionova TV, Terekhova IS. Clathrate hydrates of tetrabutylammonium and tetraisoamylammonium halides. Journal of Structural Chemistry. 2002;43:990-4.
- Babu P, Yang T, Veluswamy HP, Kumar R, Linga P. Hydrate phase equilibrium of ternary gas mixtures containing carbon dioxide, hydrogen and propane. The Journal of Chemical Thermodynamics. 2013;61:58-63.
- Park S, Lee S, Lee Y, Lee Y, Seo Y. Hydrate-based pre-combustion capture of carbon dioxide in the presence of a thermodynamic promoter and porous silica gels. International Journal of Greenhouse Gas Control. 2013;14:193-9.